12.1 Identifying The Substance Of Genes
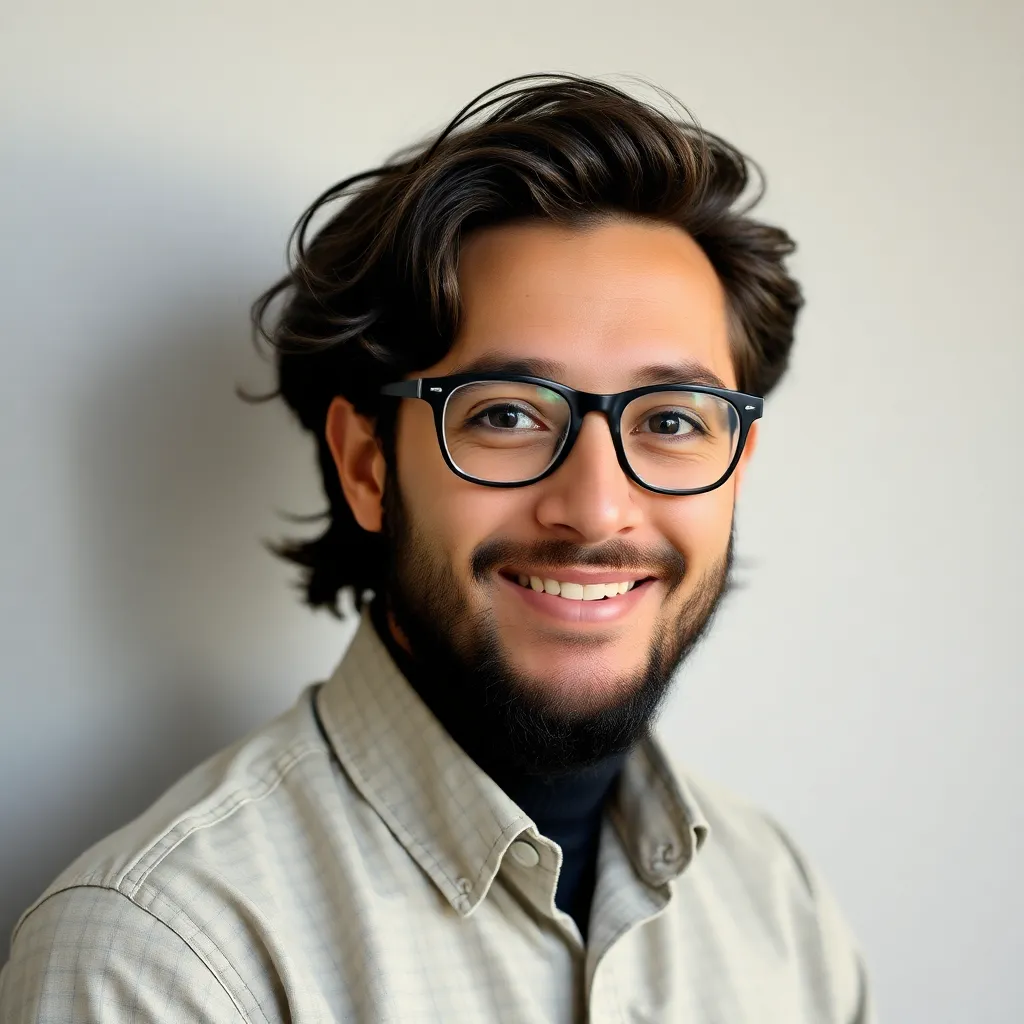
Onlines
Apr 03, 2025 · 6 min read
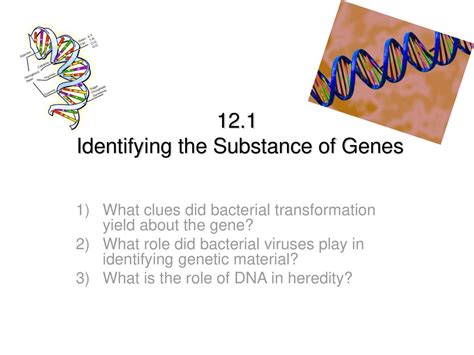
Table of Contents
12.1 Identifying the Substance of Genes: A Deep Dive into the History and Mechanisms
The question of what constitutes a gene – the fundamental unit of heredity – has captivated scientists for over a century. Unraveling the substance of genes was a monumental task, requiring decades of meticulous research, ingenious experimentation, and the convergence of multiple scientific disciplines. This journey, from initial speculation to our current sophisticated understanding, is a testament to the power of scientific inquiry. This article will explore the key historical milestones and scientific breakthroughs that led to the identification of DNA as the genetic material, delve into the structure and function of genes, and touch upon contemporary challenges and future directions in this field.
Early Speculations and the Search for the "Transforming Principle"
Before the identification of DNA, scientists were aware of the existence of genes based on observable patterns of inheritance. Gregor Mendel’s groundbreaking work in the 19th century established the fundamental principles of inheritance, but the physical nature of these heritable factors remained a mystery. The early 20th century saw the rise of cytology, the study of cells, which provided crucial visual clues. Scientists observed chromosomes, thread-like structures within the cell nucleus, that seemed to segregate during cell division in a manner consistent with Mendel’s laws. This led to the chromosome theory of inheritance, proposing that genes were located on chromosomes.
A pivotal experiment by Frederick Griffith in 1928 significantly advanced the search. Griffith's work with Streptococcus pneumoniae bacteria, famously known as the "transforming principle" experiment, demonstrated that a heritable factor could be transferred from one bacterial strain to another, transforming its properties. He showed that a heat-killed virulent strain of bacteria could transform a live, non-virulent strain into a virulent one. This suggested that some substance, capable of carrying genetic information, was responsible for this transformation.
Avery, MacLeod, and McCarty: Identifying DNA as the Transforming Principle
The identity of Griffith's "transforming principle" remained elusive until the groundbreaking work of Oswald Avery, Colin MacLeod, and Maclyn McCarty in the 1940s. They painstakingly purified the transforming substance from the heat-killed virulent bacteria, systematically eliminating different classes of biological molecules (proteins, lipids, carbohydrates, etc.). Their results consistently showed that only DNA retained its transforming ability. This experiment provided compelling evidence, although not universally accepted at the time, strongly suggesting that DNA, not protein, was the genetic material.
The Hershey-Chase Experiment: Confirmation with Bacteriophages
Further confirmation of DNA's role came from the elegant experiments of Alfred Hershey and Martha Chase in 1952. They used bacteriophages, viruses that infect bacteria, to demonstrate definitively that DNA, not protein, was the genetic material. They labeled the phage's DNA with radioactive phosphorus (³²P) and its protein coat with radioactive sulfur (³⁵S). After allowing the phages to infect bacteria, they found that the radioactive phosphorus entered the bacterial cells, while the radioactive sulfur remained outside. This conclusively showed that the phage's genetic material, responsible for directing the production of new phages, was DNA.
The Structure of DNA and the Genetic Code
With DNA established as the genetic material, the next major challenge was to determine its structure. The work of Rosalind Franklin, using X-ray diffraction techniques, provided crucial data that was instrumental in the discovery of DNA's double helix structure by James Watson and Francis Crick in 1953. This landmark achievement revealed how DNA could replicate itself accurately and store genetic information. The double helix, with its complementary base pairing (adenine with thymine, and guanine with cytosine), provided a mechanism for both accurate replication and information storage.
The subsequent cracking of the genetic code – deciphering how the sequence of nucleotides in DNA dictates the sequence of amino acids in proteins – was another monumental achievement. This understanding explained how the information encoded in DNA is translated into the functional molecules that build and regulate the organism. The central dogma of molecular biology – DNA to RNA to protein – solidified our understanding of the flow of genetic information.
Genes: Beyond Simple Definitions
Initially, the concept of a gene was relatively simple: a unit of heredity that determines a single trait. However, as our understanding deepened, the definition of a gene became significantly more nuanced. Genes are not just discrete units; they are complex regions of DNA with intricate regulatory mechanisms.
Gene Structure and Regulation:
A typical eukaryotic gene comprises several key components:
- Promoter: A region of DNA that initiates transcription, the process of creating an RNA copy of the gene.
- Exons: Coding sequences that are translated into protein.
- Introns: Non-coding sequences that are transcribed but then spliced out before translation.
- Enhancers and Silencers: Regulatory regions that can modulate the level of gene expression.
- Termination sequence: A signal that marks the end of transcription.
The process of gene expression is tightly regulated, involving various mechanisms that control when, where, and to what extent a gene is transcribed and translated. These mechanisms include transcriptional regulation, post-transcriptional regulation, translational regulation, and post-translational regulation. This intricate control ensures that genes are expressed only when and where needed, maintaining cellular homeostasis and orchestrating developmental processes.
Types of Genes and their Functions:
Genes are diverse in their functions and can be broadly categorized as:
- Protein-coding genes: These genes encode the instructions for building proteins, the workhorses of the cell.
- Non-coding RNA genes: These genes transcribe RNA molecules that do not get translated into proteins but play crucial roles in gene regulation, translation, and other cellular processes. Examples include ribosomal RNA (rRNA), transfer RNA (tRNA), and microRNAs (miRNAs).
- Regulatory genes: These genes control the expression of other genes, often acting as switches or dials that modulate the activity of entire pathways.
Challenges and Future Directions
Despite our significant advances, many aspects of gene function remain poorly understood. Some of the current challenges and future research directions include:
- Epigenetics: The study of heritable changes in gene expression that do not involve alterations in the DNA sequence itself. Epigenetic modifications, such as DNA methylation and histone modification, play significant roles in gene regulation and disease development.
- Gene editing technologies: CRISPR-Cas9 and other gene editing techniques are revolutionizing our ability to modify genes, offering potential cures for genetic diseases and advancements in biotechnology. However, ethical considerations surrounding gene editing remain a major concern.
- Systems biology: The study of complex biological systems as integrated networks, allowing researchers to investigate the interactions between genes and their regulatory elements in a holistic manner.
- Genomics and personalized medicine: Advances in genomics, the study of entire genomes, are paving the way for personalized medicine, tailoring treatments to an individual's unique genetic makeup.
Conclusion
The identification of the substance of genes, a journey spanning over a century, stands as a triumph of scientific investigation. From the initial observations of inheritance patterns to the elucidation of DNA's structure and the cracking of the genetic code, this journey has revolutionized our understanding of life itself. While we have made tremendous progress, many unanswered questions remain, demanding further research and innovation. The future of genetics holds immense promise, with potential breakthroughs in gene therapy, personalized medicine, and our understanding of complex biological systems. The continuous exploration of the intricate world of genes is essential for advancing human health, addressing global challenges, and pushing the boundaries of our knowledge about life itself. The journey of understanding genes continues, and the next chapter is sure to be equally exciting and revealing.
Latest Posts
Latest Posts
-
Good Password Management Include Which Three Of The Following
Apr 03, 2025
-
Describe These People Using Expressions With Avoir
Apr 03, 2025
-
Continuous Phenotypic Variation Is Observed When
Apr 03, 2025
-
Circle The Correct Choice Within The Parenthesis For 1 18
Apr 03, 2025
-
Amoeba Sisters Video Recap Genetic Drift
Apr 03, 2025
Related Post
Thank you for visiting our website which covers about 12.1 Identifying The Substance Of Genes . We hope the information provided has been useful to you. Feel free to contact us if you have any questions or need further assistance. See you next time and don't miss to bookmark.