A Common Alkene Starting Material Is Shown Below
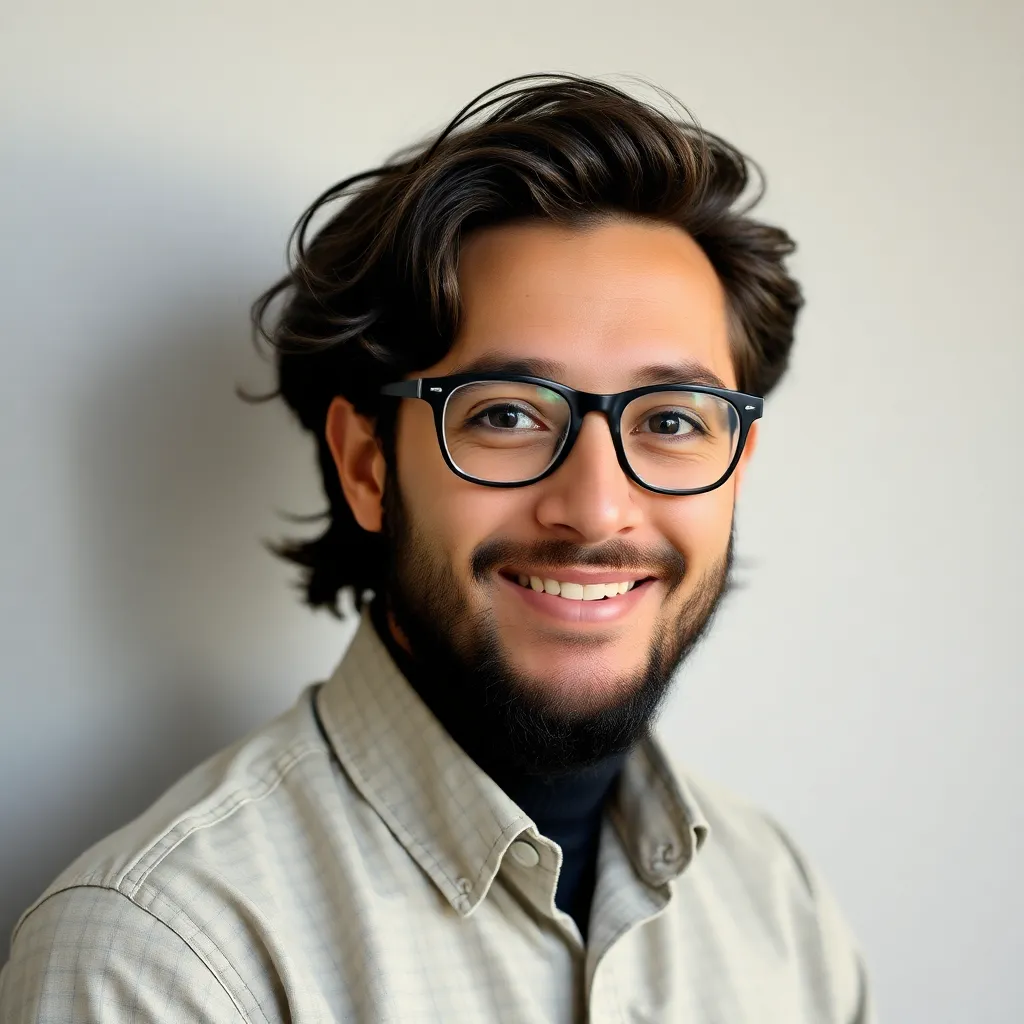
Onlines
Apr 03, 2025 · 6 min read
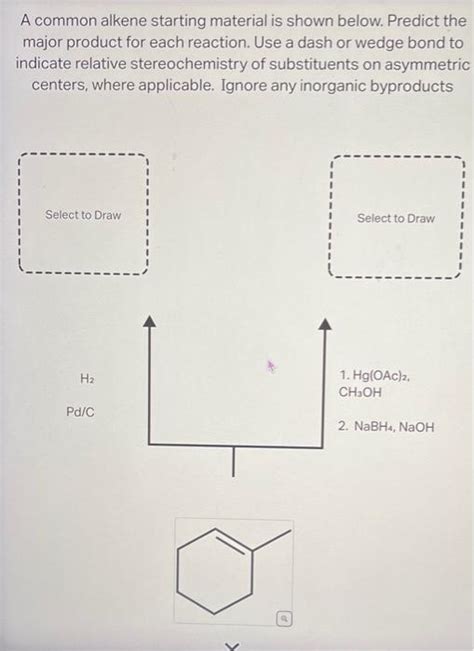
Table of Contents
A Common Alkene Starting Material: Exploring the Versatility of Ethylene
Ethylene, systematically named ethene, is arguably the most fundamental and ubiquitous alkene starting material in the chemical industry. Its simple structure—a carbon-carbon double bond flanked by two hydrogen atoms (CH₂=CH₂) — belies its extraordinary reactivity and versatility. This seemingly simple molecule serves as a cornerstone for the synthesis of a vast array of commercially important compounds, impacting numerous aspects of modern life. This article delves into the properties, production, and diverse applications of ethylene, highlighting its pivotal role as a building block for countless products.
Understanding Ethylene's Chemical Properties
Ethylene's unique reactivity stems from the presence of the carbon-carbon double bond. This π (pi) bond, formed by the lateral overlap of p-orbitals, is significantly weaker than the σ (sigma) bond formed by the head-on overlap of sp² hybridized orbitals. This difference in bond strength makes the π bond susceptible to attack by electrophiles, initiating a wide array of electrophilic addition reactions.
Key Chemical Properties:
- Unsaturation: The presence of the double bond is the defining characteristic of ethylene, classifying it as an unsaturated hydrocarbon. This unsaturation is the key to its reactivity.
- Planar Geometry: The carbon atoms in ethylene are sp² hybridized, resulting in a trigonal planar geometry around each carbon atom. All atoms lie in the same plane.
- Polarizability: While nonpolar overall, the electron density in the π bond is relatively mobile, making ethylene susceptible to polarization by other molecules or ions. This polarizability contributes to its reactivity in both polar and nonpolar environments.
- Electrophilic Addition: This is the dominant reaction type for ethylene. Electrophiles (electron-deficient species) readily attack the π bond, leading to the formation of new C-X bonds (where X is the electrophile). Examples include the addition of halogens (e.g., Cl₂, Br₂), hydrogen halides (e.g., HCl, HBr), and water (in the presence of an acid catalyst).
- Polymerization: Ethylene's ability to undergo addition polymerization is of paramount importance. Under appropriate conditions, numerous ethylene molecules can link together to form long chains, resulting in the formation of polyethylene, one of the world's most widely used plastics.
- Oxidation: Ethylene can be oxidized to produce a variety of oxygenated compounds, including ethylene oxide, acetaldehyde, and acetic acid. These oxidation reactions often involve catalysts and controlled conditions.
Industrial Production of Ethylene: Steam Cracking
The overwhelming majority of industrial ethylene production relies on the steam cracking process. This process involves heating a hydrocarbon feedstock, typically ethane or naphtha, to extremely high temperatures (800-900°C) in the presence of steam. The high temperature breaks down the larger hydrocarbon molecules into smaller fragments, including ethylene.
Steps Involved in Steam Cracking:
- Preheating: The hydrocarbon feedstock is preheated to a moderate temperature.
- Rapid Heating: The preheated feedstock is rapidly heated to the cracking temperature in a furnace. The rapid heating minimizes unwanted side reactions.
- Quenching: The hot cracking products are rapidly cooled (quenched) to prevent further reactions.
- Separation: The quenched products are then separated using a series of distillation columns to isolate ethylene from other components such as propylene, butenes, and aromatics.
The efficiency of steam cracking depends on several factors, including the type of feedstock, the temperature and pressure in the furnace, and the residence time of the hydrocarbons in the reactor. Optimizing these parameters is crucial for maximizing ethylene yield and minimizing the formation of undesirable byproducts.
Other methods for ethylene production exist, but they are less significant on an industrial scale. These include the dehydration of ethanol and the catalytic dehydrogenation of ethane.
Diverse Applications of Ethylene and its Derivatives
The versatility of ethylene as a starting material is evident in the vast array of products derived from it. Its applications span various industries, including plastics, textiles, and pharmaceuticals.
1. Polyethylene: The most significant application of ethylene is its polymerization to produce polyethylene (PE). This versatile plastic is used in countless applications, from plastic bags and films to bottles and pipes. Different types of polyethylene, such as high-density polyethylene (HDPE) and low-density polyethylene (LDPE), are produced by varying the polymerization conditions, leading to different physical properties.
2. Ethylene Oxide: The oxidation of ethylene yields ethylene oxide, a crucial intermediate in the production of ethylene glycol. Ethylene glycol is a primary component of antifreeze and is also used in the production of polyester fibers. Ethylene oxide itself finds applications as a sterilizing agent and in the production of various other chemicals.
3. Ethylene Dichloride (EDC): The reaction of ethylene with chlorine produces ethylene dichloride, a key intermediate in the production of vinyl chloride monomer (VCM). VCM is then polymerized to produce polyvinyl chloride (PVC), a widely used plastic in construction, packaging, and other industries.
4. Ethylbenzene: The alkylation of benzene with ethylene yields ethylbenzene, a precursor to styrene. Styrene is then polymerized to produce polystyrene, a versatile plastic used in packaging, insulation, and various other applications.
5. Ethanol: Ethylene can be hydrated to produce ethanol, an important industrial solvent and fuel additive. Ethanol is also used in the production of beverages and pharmaceuticals.
6. Acetaldehyde: The oxidation of ethylene under specific conditions produces acetaldehyde, a valuable intermediate used in the production of acetic acid, ethanol, and other chemicals.
7. Acetic Acid: Acetic acid, a ubiquitous chemical, can be produced from ethylene through several routes, often involving the intermediacy of acetaldehyde. It is used extensively in the food industry as a preservative and in the production of various chemical products.
Environmental Considerations and Sustainable Practices
The large-scale production and use of ethylene and its derivatives raise important environmental considerations. The production process, particularly steam cracking, requires significant energy input and can generate greenhouse gas emissions. Furthermore, the disposal of polyethylene and other plastic materials presents a major environmental challenge due to their persistence in the environment.
Sustainable Practices and Future Directions:
- Improving Energy Efficiency: Research focuses on developing more energy-efficient steam cracking processes and alternative production methods, such as bio-based ethylene production from biomass.
- Recycling and Waste Management: Improved recycling technologies and waste management strategies are essential to minimize the environmental impact of plastic waste.
- Developing Bio-Based Alternatives: The use of renewable resources as feedstocks for ethylene production is gaining traction, offering a more sustainable alternative to fossil-fuel-based methods.
- Exploring Catalytic Oxidation and other greener reaction pathways: Research is looking at more selective catalysts that can minimize unwanted byproducts and improve the atom economy of ethylene conversion.
Conclusion
Ethylene, despite its simple molecular structure, stands as a cornerstone of the modern chemical industry. Its versatility and reactivity have driven the development of a wide array of essential products that impact our daily lives. However, the sustainability of ethylene production and the management of its derivatives remain crucial challenges. Continued research and innovation are needed to minimize the environmental impact of ethylene-based technologies while exploring sustainable alternatives and developing more environmentally responsible practices. The future of ethylene hinges on addressing these environmental concerns to ensure a balance between industrial progress and environmental protection. This ongoing exploration of improved production methods and waste management solutions will define the role of ethylene in a future focused on sustainability and responsible chemical manufacturing.
Latest Posts
Latest Posts
-
Nos Indica El Lugar Del Teatro
Apr 04, 2025
-
Appc Lesson 1 4 Homework Answer Key
Apr 04, 2025
-
Supervisors May Use A Ta To Monitor Your Work
Apr 04, 2025
-
Marketers Dont Track Customer Evaluations Because
Apr 04, 2025
-
A Distance Divided By A Time Is Called A
Apr 04, 2025
Related Post
Thank you for visiting our website which covers about A Common Alkene Starting Material Is Shown Below . We hope the information provided has been useful to you. Feel free to contact us if you have any questions or need further assistance. See you next time and don't miss to bookmark.