A Typical White Dwarf Is _________.
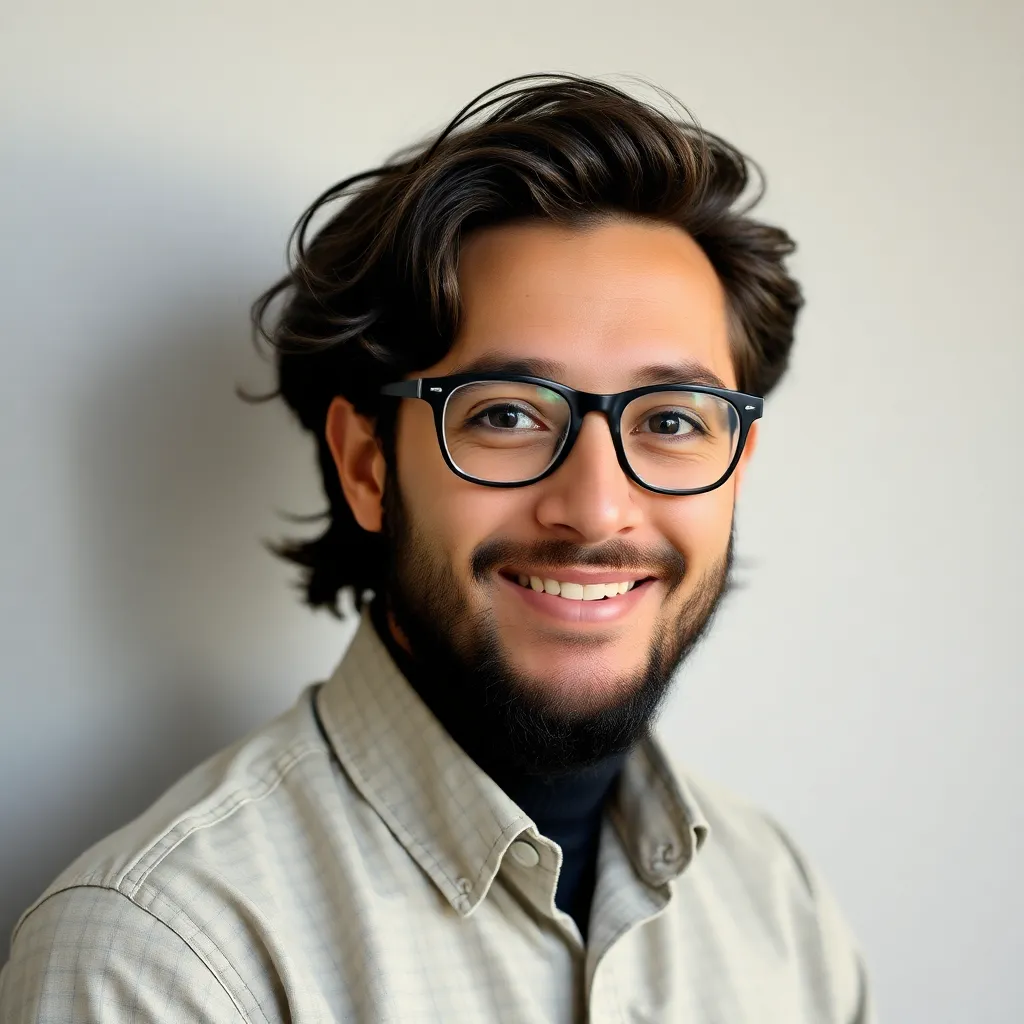
Onlines
May 11, 2025 · 7 min read
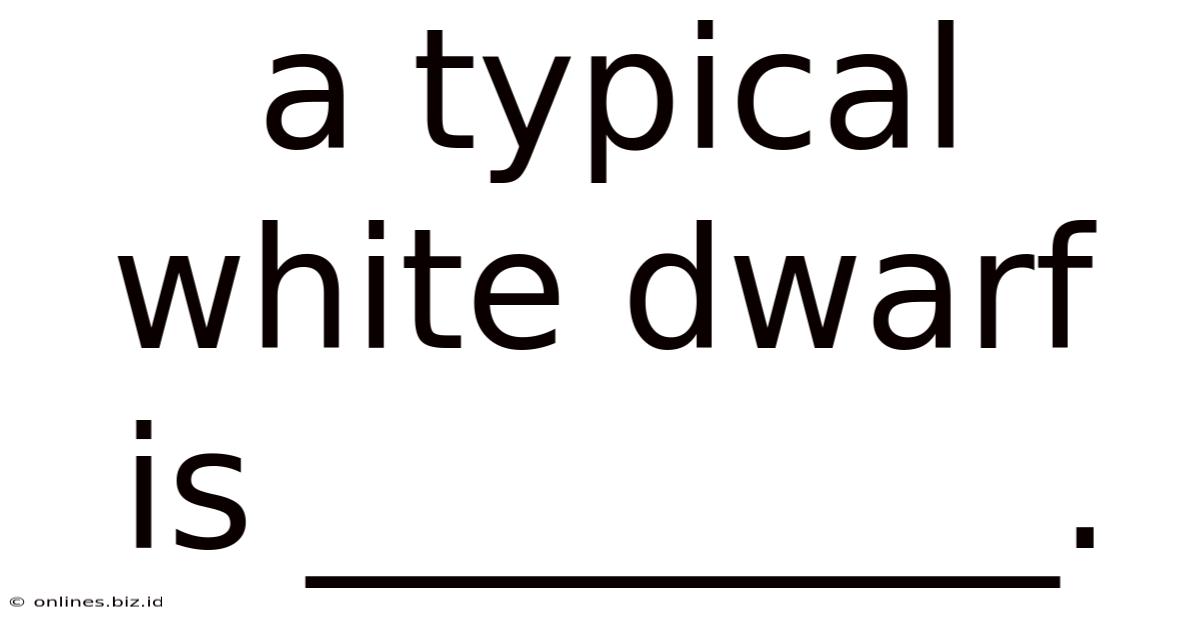
Table of Contents
A Typical White Dwarf Is... Surprisingly Complex!
A typical white dwarf is a dense, Earth-sized remnant of a star that has exhausted its nuclear fuel. This simple statement, however, belies the incredible complexity and fascinating physics hidden within these stellar corpses. Understanding what constitutes a "typical" white dwarf requires delving into their formation, composition, evolution, and surprising diversity. While they might seem uniform at first glance, white dwarfs exhibit a remarkable range of properties, challenging our understanding of stellar evolution and the universe itself.
The Formation of a White Dwarf: The End of a Star's Life
The journey to becoming a white dwarf begins with a star much like our Sun – a main-sequence star fueled by hydrogen fusion in its core. For billions of years, this fusion process generates outward pressure that counteracts the inward pull of gravity. However, this delicate balance eventually ends. Once the star's hydrogen fuel is depleted, the core contracts and heats up, initiating helium fusion. This stage, known as the red giant phase, sees the star expand dramatically, engulfing its inner planets.
The fate of the star beyond this point depends on its initial mass. Stars with masses up to around eight times that of our Sun will eventually exhaust their nuclear fuel, unable to fuse heavier elements like carbon and oxygen. The outer layers of the star are then expelled into space, creating a beautiful planetary nebula. What remains is the core, now incredibly dense and hot – a white dwarf.
The Composition: Carbon and Oxygen, Mostly
A typical white dwarf is primarily composed of carbon and oxygen. These elements are the ashes of helium fusion that dominated the star's later life. The extreme density of a white dwarf arises from the electron degeneracy pressure. This is a quantum mechanical effect where electrons are packed so tightly together that they resist further compression, preventing the star from collapsing further under its own gravity. This pressure supports the white dwarf against gravitational collapse, and it's what gives white dwarfs their amazing density.
Trace Elements and Variations: Not So Typical After All
While carbon and oxygen dominate, a typical white dwarf can also contain trace amounts of other elements, depending on the star's initial mass and evolutionary path. These trace elements can include neon, magnesium, and even heavier elements. The precise abundances of these elements provide valuable clues about the star's progenitor and its evolutionary history.
Further adding to the complexity, not all white dwarfs are created equal. Some might have a helium-rich atmosphere, while others might be predominantly carbon-oxygen white dwarfs. The differences in composition reflect the variations in the progenitor star's mass and the efficiency of its nuclear burning processes.
The Physics of a White Dwarf: Degeneracy and Density
The physics of white dwarfs is governed by the principles of quantum mechanics and general relativity. The electron degeneracy pressure mentioned earlier is a crucial element. This pressure increases dramatically as the density increases, preventing further gravitational collapse. This is fundamentally different from the pressure support in main-sequence stars, which relies on the thermal pressure generated by nuclear fusion.
The density of a white dwarf is astonishingly high. A teaspoonful of white dwarf material would weigh several tons on Earth! This immense density is a direct consequence of the electron degeneracy pressure, squeezing the matter into a highly compact state. This density also dictates other properties of white dwarfs, like their surface gravity and their cooling rate.
The Chandrasekhar Limit: A Critical Mass
The maximum mass a white dwarf can attain without collapsing further is known as the Chandrasekhar limit, approximately 1.4 times the mass of our Sun. Beyond this limit, the electron degeneracy pressure is insufficient to counteract the gravitational pull, and the white dwarf will collapse into a neutron star or even a black hole, depending on its mass. This limit is a crucial factor in understanding the evolution of stars and the formation of compact objects.
The Evolution of a White Dwarf: Cooling and Fading
White dwarfs are not static objects; they gradually cool and fade over incredibly long timescales. As a white dwarf cools, its luminosity diminishes, and it transitions through different stages of its evolutionary path. The initial temperature of a white dwarf can be incredibly high, but over trillions of years, it slowly cools to a cold, dark remnant.
The cooling rate of a white dwarf depends on several factors, including its initial mass, composition, and the presence of any remaining magnetic fields. By studying the cooling rates of white dwarfs, astronomers can learn more about their age, composition, and the physics of dense matter.
Cooling Curves and Age Determination
Astronomers use observations of white dwarf cooling curves to estimate their ages. These curves, which plot the luminosity of a white dwarf against its temperature, provide valuable constraints on the age of the stellar population in which they reside. This information is crucial for understanding galactic evolution and the formation of stars.
Observing White Dwarfs: Unveiling Their Secrets
White dwarfs, though faint, are relatively common in our galaxy. Their observation provides crucial information about stellar evolution, galactic structure, and the physics of dense matter. Astronomers use various techniques to study white dwarfs, including:
- Photometry: Measuring the brightness of white dwarfs to determine their luminosity and temperature.
- Spectroscopy: Analyzing the light from white dwarfs to determine their composition and atmospheric characteristics.
- Astrometry: Precisely measuring the positions of white dwarfs to study their movements and gravitational interactions.
These observations, combined with theoretical models, provide a detailed picture of white dwarf properties, evolution, and the processes that shape the cosmos.
Studying White Dwarf Atmospheres: Clues to Composition and Evolution
Analyzing the atmospheres of white dwarfs is crucial in understanding their composition. The presence of certain elements in the atmosphere provides clues about the star's evolutionary history and the processes that shaped its composition. For instance, the detection of heavy elements like metals in some white dwarf atmospheres suggests accretion of material from a companion star or planet.
White Dwarfs and Binary Systems: Accretion and Novae
Many white dwarfs are found in binary systems, orbiting another star. In such systems, the white dwarf can accrete material from its companion star. This accretion process can lead to the formation of an accretion disk around the white dwarf, and eventually, to thermonuclear explosions known as novae.
These novae are dramatic events that temporarily increase the white dwarf's luminosity by many orders of magnitude. The study of novae provides important insights into the physics of accretion, thermonuclear reactions, and the evolution of binary systems containing white dwarfs.
Type Ia Supernovae: The Ultimate Fate of Some White Dwarfs
In some binary systems containing white dwarfs, the accretion of matter can ultimately lead to the white dwarf exceeding the Chandrasekhar limit. This results in a catastrophic explosion known as a Type Ia supernova, one of the brightest events in the universe. Type Ia supernovae are used as "standard candles" in cosmology, helping astronomers to measure vast distances and the expansion rate of the universe. This highlights the crucial role white dwarfs play in understanding cosmological scales and the evolution of the entire universe.
The Future of White Dwarf Research: Unanswered Questions
Despite significant progress in our understanding of white dwarfs, many mysteries remain. Scientists are actively working to address questions such as:
- The precise mechanisms responsible for the cooling rates of white dwarfs: This involves improving our understanding of the physics of dense matter and the transport of heat within white dwarfs.
- The origin and evolution of white dwarf magnetic fields: Understanding the role of magnetic fields in white dwarf evolution and their potential impact on their cooling rates.
- The detailed composition and structure of white dwarf interiors: This requires developing more advanced theoretical models and observational techniques.
The answers to these questions will refine our understanding of white dwarfs, stellar evolution, and the universe at large. The study of these seemingly simple stellar remnants is a dynamic field of astrophysical research, promising further exciting discoveries in the years to come. Therefore, the seemingly simple statement “a typical white dwarf is a dense, Earth-sized remnant of a star” only scratches the surface of their rich complexity and fascinating role in shaping the universe.
Latest Posts
Latest Posts
-
The Act Of Managing Your Emotions After You Ve Assessed Them
May 12, 2025
-
Chapter 1 The Catcher In The Rye
May 12, 2025
-
Correctly Label The Anatomical Features Of A Neuromuscular Junction
May 12, 2025
-
Why Is Oil A Dominant Medium In Easel Painting
May 12, 2025
-
Explain Why The Benzocaine Precipitates Out During Neutralization
May 12, 2025
Related Post
Thank you for visiting our website which covers about A Typical White Dwarf Is _________. . We hope the information provided has been useful to you. Feel free to contact us if you have any questions or need further assistance. See you next time and don't miss to bookmark.