Ac Theory Level 3 Lesson 3
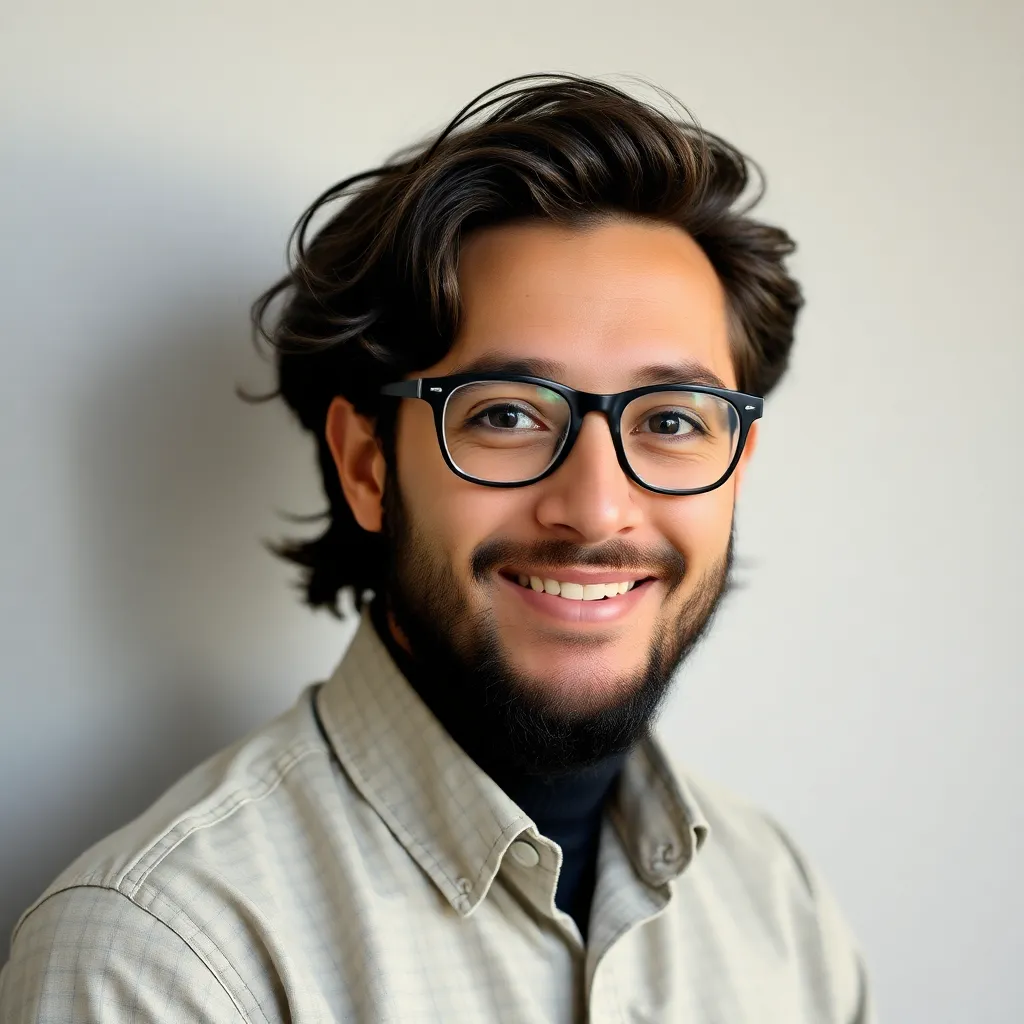
Onlines
Apr 02, 2025 · 7 min read
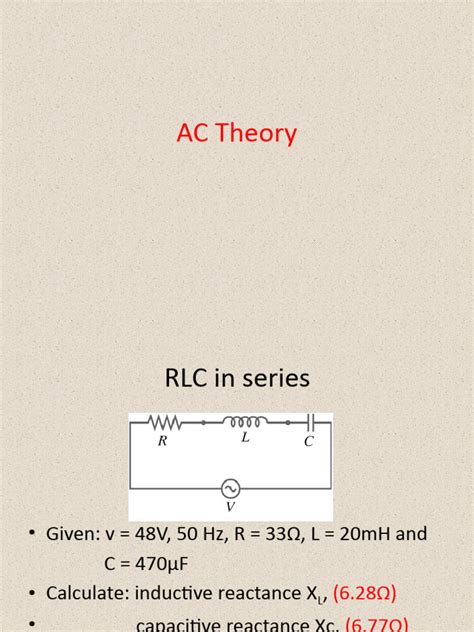
Table of Contents
AC Theory Level 3, Lesson 3: Advanced Power Factor Correction and Three-Phase Systems
This lesson delves into the complexities of power factor correction (PFC) and three-phase AC systems, building upon the foundational knowledge gained in previous AC theory levels. We'll explore advanced PFC techniques, analyze three-phase power systems, and examine the implications of unbalanced loads. This comprehensive guide aims to provide a clear and thorough understanding of these crucial aspects of alternating current theory.
Advanced Power Factor Correction Techniques
Power factor correction (PFC) is crucial for efficient electrical systems. While earlier lessons covered the basics of PFC using simple capacitor banks, advanced applications require more sophisticated approaches to address complex loads and varying power demands.
1. Stepped Power Factor Correction
Simple capacitor banks provide a fixed level of PFC. However, load variations necessitate a more dynamic solution. Stepped PFC involves using multiple capacitor banks, switched in or out of the circuit depending on the load's power factor. This allows for precise adjustment to maintain a near-unity power factor across a range of operating conditions.
Advantages:
- Improved efficiency: Maintains a high power factor across a wider range of loads.
- Reduced losses: Minimizes energy waste caused by low power factors.
- Cost-effective: More efficient than continuously adjusting a single large capacitor bank.
Disadvantages:
- Increased complexity: Requires more sophisticated switching mechanisms and control systems.
- Potential for switching transients: Switching large capacitor banks can create voltage spikes or transients that need careful management.
2. Active Power Factor Correction (APFC)
Active PFC utilizes electronic circuitry to dynamically adjust the current waveform, ensuring it's in phase with the voltage waveform. Unlike passive methods that rely on fixed components, APFC employs power electronic devices, such as IGBTs (Insulated Gate Bipolar Transistors) or MOSFETs (Metal-Oxide-Semiconductor Field-Effect Transistors), to shape the current waveform. This allows for precise and continuous control of the power factor, resulting in significantly improved efficiency.
Advantages:
- Excellent power factor correction: Achieves near-unity power factor even with highly nonlinear loads.
- Improved harmonic reduction: Minimizes harmonic distortion in the power system.
- Enhanced efficiency: Reduces energy losses and improves overall system efficiency.
Disadvantages:
- Higher cost: More expensive than passive PFC methods due to the complex circuitry involved.
- Increased complexity: Requires sophisticated control algorithms and power electronics expertise.
- Potential for increased harmonic distortion (if not properly designed): While designed to reduce harmonics, improper design can exacerbate the problem.
3. Hybrid Power Factor Correction
Hybrid PFC combines the advantages of both passive and active PFC methods. A passive PFC stage provides the bulk of the correction, while an active PFC stage handles fine-tuning and addresses remaining harmonic distortions. This approach balances the cost and performance benefits, offering a cost-effective solution for many applications.
Advantages:
- Cost-effective: Balances the cost of passive and active components.
- High power factor: Combines the benefits of both passive and active PFC techniques.
- Improved harmonic reduction: Addresses harmonic distortions effectively.
Disadvantages:
- Increased complexity: Involves a more intricate system design than either passive or active PFC alone.
- Requires careful component selection and system integration: Proper design and integration are critical for optimal performance.
Three-Phase AC Systems
Three-phase AC systems are the backbone of most industrial and high-power applications. They offer significant advantages over single-phase systems, including higher power capacity, smoother power delivery, and improved efficiency.
1. Three-Phase Voltage and Current Relationships
Three-phase systems employ three sinusoidal voltages, 120 degrees out of phase with each other. This phase displacement is critical for balanced operation and efficient power transfer. The relationship between line and phase voltages, as well as line and phase currents, is fundamental to understanding three-phase systems' behavior. In a balanced system, the line voltage is √3 times the phase voltage, and the line current is equal to the phase current.
2. Types of Three-Phase Connections
Two main types of three-phase connections are prevalent: star (wye) and delta. The choice of connection impacts the voltage and current relationships and affects the system's overall characteristics.
-
Star (Wye) Connection: In a star connection, one end of each phase winding is connected to a common neutral point. The line voltage is √3 times the phase voltage, and the line current equals the phase current.
-
Delta Connection: In a delta connection, the three phase windings are connected in a closed loop. The line voltage equals the phase voltage, and the line current is √3 times the phase current.
3. Power Calculations in Three-Phase Systems
Calculating power in three-phase systems is slightly more complex than in single-phase systems due to the multiple phases. The total power is the sum of the power in each phase. In a balanced system, the calculations are simplified, and total power can be easily calculated using formulas involving line voltage, line current, and power factor.
4. Unbalanced Three-Phase Systems
Unbalanced three-phase systems arise when the load on each phase is not equal. This imbalance leads to several undesirable effects, including:
- Unequal phase currents: Leads to higher currents in some phases, potentially exceeding the capacity of the conductors.
- Neutral current: An unbalanced load creates a neutral current, which can be problematic in some systems.
- Harmonic distortion: Unbalanced loads can introduce harmonic distortion into the power system.
- Reduced efficiency: Power losses are increased due to the imbalance.
5. Power Factor Correction in Three-Phase Systems
Power factor correction is equally crucial in three-phase systems as it is in single-phase systems. However, the implementation differs due to the multiple phases. Capacitor banks or active PFC devices are used to compensate for the reactive power and improve the overall power factor. The placement and sizing of PFC components are critical for balancing the system and achieving optimal efficiency.
Advanced Topics and Applications
This section briefly explores some advanced aspects of AC theory relevant to Level 3 studies:
1. Harmonic Analysis
Harmonic analysis plays a vital role in understanding and mitigating the effects of nonlinear loads on power systems. Nonlinear loads generate harmonic currents, which can cause various problems, including overheating of equipment, interference with communication systems, and increased power losses. Techniques for harmonic analysis, such as Fourier analysis, are essential tools for diagnosing and solving harmonic-related issues.
2. Power System Transient Analysis
Power system transient analysis focuses on understanding how power systems respond to sudden changes or disturbances, such as faults or switching operations. These analyses are critical for designing robust and reliable power systems that can withstand transient events without causing damage or interruptions.
3. Power System Protection
Effective power system protection is essential for ensuring the safety and reliability of electrical systems. Protection systems use various devices and techniques to detect faults and isolate affected sections of the system, minimizing the impact of disturbances and preventing damage to equipment.
4. Power Quality
Power quality refers to the characteristics of the electrical power supply that affect the performance of equipment and systems. Maintaining good power quality involves minimizing voltage fluctuations, harmonic distortions, and other disturbances that can compromise the reliability and efficiency of electrical systems.
5. Applications of Three-Phase Systems in Industrial Settings
Three-phase systems are ubiquitous in industrial settings due to their ability to power large motors, industrial machinery, and other high-power equipment. Understanding the characteristics of three-phase systems is critical for designing and maintaining efficient and reliable industrial power systems.
Conclusion
This lesson provided an advanced look at power factor correction and three-phase AC systems. Mastering these concepts is crucial for electrical engineers and technicians working with high-power applications. From understanding advanced PFC techniques to analyzing the intricacies of three-phase systems and dealing with unbalanced loads, this knowledge forms a solid foundation for further studies in power systems engineering and related fields. Further exploration of the advanced topics mentioned will enhance your understanding and expertise in the field of alternating current theory. Remember to always prioritize safety and adhere to proper electrical codes and practices when working with AC systems.
Latest Posts
Latest Posts
-
Middle East And South Asia Ii Unit Test
Apr 03, 2025
-
Chapter 2 Pedagogy Of The Oppressed
Apr 03, 2025
-
Which Is Not Recommended When Giving Feedback
Apr 03, 2025
-
A Simple Elevator Ride Can Teach
Apr 03, 2025
-
Penelope 1 Of 1 Muestra Sus Fotos A Mi
Apr 03, 2025
Related Post
Thank you for visiting our website which covers about Ac Theory Level 3 Lesson 3 . We hope the information provided has been useful to you. Feel free to contact us if you have any questions or need further assistance. See you next time and don't miss to bookmark.