Art-labeling Activity Structure Of The Nucleic Acids Dna And Rna
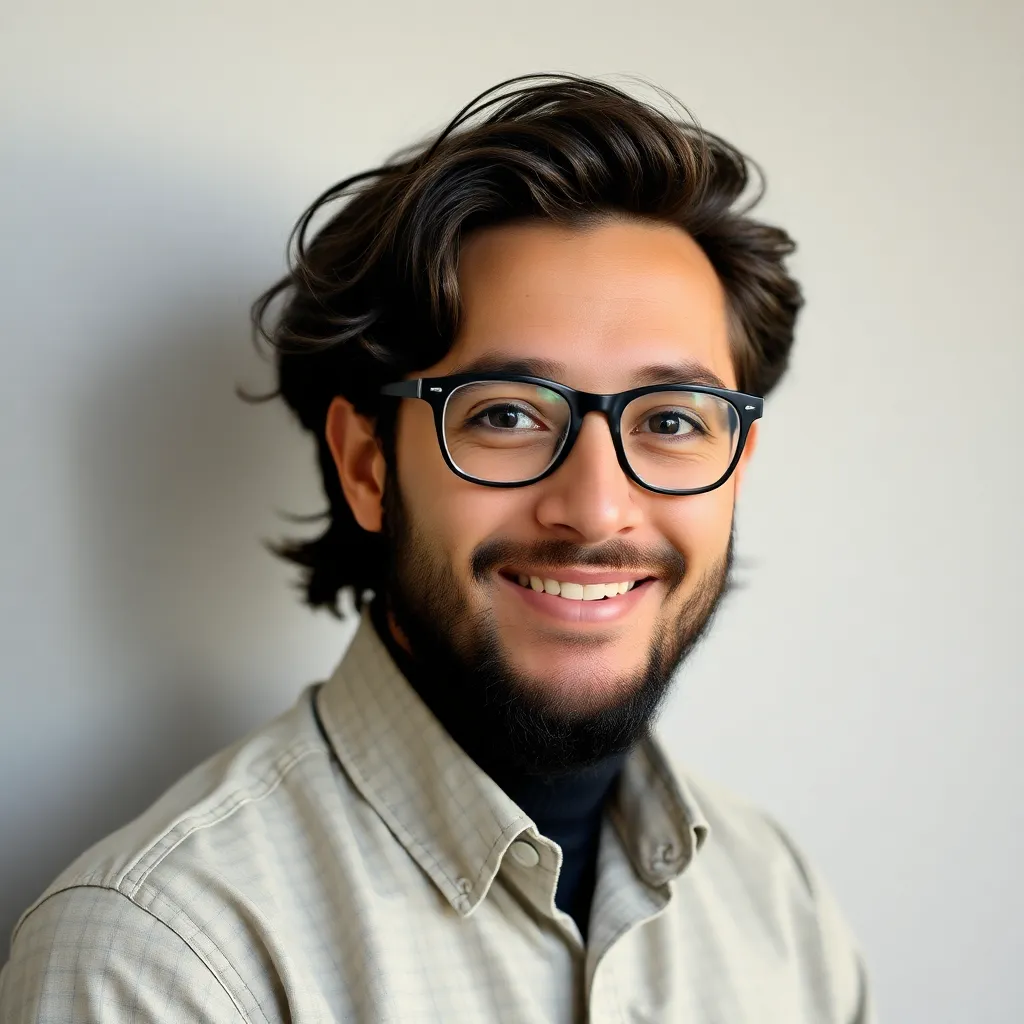
Onlines
Apr 27, 2025 · 6 min read

Table of Contents
Art-Labeling Activity Structure of the Nucleic Acids DNA and RNA
The intricate dance of life hinges on the elegant structure and dynamic activity of nucleic acids, primarily DNA and RNA. Understanding their structure is crucial to grasping their roles in genetic information storage, transfer, and expression. This article delves deep into the art of labeling nucleic acids, exploring the diverse techniques used to visualize and analyze their structures and functions. We will explore various labeling methods, their applications, and the crucial implications for understanding the complexities of life itself.
The Fundamental Architecture: DNA and RNA
Before delving into labeling techniques, let's revisit the foundational structures of DNA and RNA. Both are polymers composed of nucleotide monomers. Each nucleotide comprises three components:
- A nitrogenous base: Adenine (A), Guanine (G), Cytosine (C), and Thymine (T) in DNA; Uracil (U) replaces Thymine in RNA.
- A five-carbon sugar: Deoxyribose in DNA, ribose in RNA.
- A phosphate group: Links nucleotides together to form the polynucleotide chain.
DNA: The Double Helix
DNA's iconic double helix structure, discovered by Watson and Crick, is fundamental to its function as the primary repository of genetic information. Two antiparallel polynucleotide strands twist around each other, held together by hydrogen bonds between complementary base pairs (A with T, and G with C). This double helix structure allows for efficient storage and replication of genetic information.
RNA: The Versatile Single Strand
Unlike DNA's stable double helix, RNA typically exists as a single-stranded molecule, although it can fold into complex secondary and tertiary structures due to intramolecular base pairing. This structural flexibility allows RNA to perform diverse roles, including:
- Messenger RNA (mRNA): Carries genetic information from DNA to ribosomes for protein synthesis.
- Transfer RNA (tRNA): Delivers specific amino acids to the ribosome during translation.
- Ribosomal RNA (rRNA): A structural and catalytic component of ribosomes.
- Small nuclear RNA (snRNA): Involved in RNA processing in the nucleus.
- MicroRNA (miRNA): Regulates gene expression by binding to mRNA molecules.
The Art of Labeling: Visualizing Nucleic Acids
Labeling nucleic acids is a cornerstone of molecular biology research. It allows researchers to visualize, quantify, and manipulate these molecules, providing crucial insights into their structure, function, and interactions. Various methods exist, each with its strengths and limitations:
1. Radioactive Isotopes: The Classic Approach
Historically, radioactive isotopes like ³²P (phosphorus-32) were widely used to label nucleic acids. ³²P incorporates into the phosphate backbone during DNA or RNA synthesis. Detection is achieved through autoradiography, revealing the location and quantity of labeled nucleic acids. While highly sensitive, this method poses safety concerns due to radioactivity and has largely been replaced by non-radioactive alternatives.
2. Fluorescent Labels: Brightness and Specificity
Fluorescent labels, such as fluorescein isothiocyanate (FITC) and rhodamine, are widely used due to their high sensitivity and versatility. These dyes bind to specific nucleotide bases or incorporate into the nucleic acid backbone. Fluorescent microscopy allows visualization of labeled nucleic acids in cells or tissues. Different fluorescent dyes can be used simultaneously to label multiple targets, enabling the study of complex interactions. Examples include:
- Ethidium bromide: Intercalates between DNA base pairs, causing fluorescence upon UV excitation. Used in gel electrophoresis to visualize DNA.
- SYBR Green: Another intercalating dye, less mutagenic than ethidium bromide.
- Fluorescently labeled nucleotides: Incorporated during DNA or RNA synthesis, enabling tracking of newly synthesized molecules.
3. Biotin and Digoxigenin: High Affinity and Versatile Detection
Biotin and digoxigenin are haptens, small molecules that bind with high affinity to specific antibodies or streptavidin. These molecules can be coupled to nucleotides or oligonucleotides and incorporated into nucleic acids. Detection is achieved using labeled antibodies or streptavidin, providing high sensitivity and specificity. This system allows for a range of detection strategies, including colorimetric, chemiluminescent, and fluorescent methods.
4. Chromogenic Labels: Visible Colorimetric Detection
Chromogenic labels, such as horseradish peroxidase (HRP) or alkaline phosphatase (AP), produce a colored product upon enzymatic reaction with a substrate. These enzymes can be coupled to antibodies or streptavidin, providing a visible signal for labeled nucleic acids. This method is widely used in techniques like Southern and Northern blotting.
5. Affinity-Based Labeling: Targeting Specific Sequences
Affinity-based labeling utilizes probes or antibodies that specifically bind to target sequences within nucleic acids. These probes can be labeled with various reporters, such as fluorescent dyes or enzymes, allowing visualization and detection of specific sequences within a complex mixture. This approach is widely used in techniques like in situ hybridization (ISH), fluorescence in situ hybridization (FISH), and microarray analysis.
Applications of Nucleic Acid Labeling
The art of labeling nucleic acids finds applications across numerous fields of biology and medicine:
1. Gene Expression Analysis: Quantifying mRNA Levels
Labeling mRNA allows quantification of gene expression levels in different cells or tissues. Techniques such as Northern blotting and quantitative real-time PCR (qPCR) rely on labeled probes to detect and quantify specific mRNA transcripts.
2. Chromosomal Analysis: Visualizing Genomic Structure
Fluorescent in situ hybridization (FISH) uses fluorescently labeled probes to visualize specific chromosomal regions or genes. This technique is crucial for diagnosing chromosomal abnormalities and studying genomic organization.
3. DNA Sequencing: Determining Nucleotide Order
Next-generation sequencing (NGS) technologies often utilize labeled nucleotides to identify the order of bases in DNA. Fluorescently labeled nucleotides are incorporated during DNA synthesis, allowing detection of each base as it is added.
4. Genomics and Proteomics: Exploring Cellular Interactions
Labeling nucleic acids plays a key role in genomics and proteomics, allowing researchers to study gene regulation, protein-DNA interactions, and other cellular processes. Techniques like chromatin immunoprecipitation (ChIP) utilize labeled antibodies to isolate and identify DNA regions bound by specific proteins.
5. Forensic Science: DNA Fingerprinting and Identification
Labeling techniques are fundamental to forensic science, allowing for DNA fingerprinting and individual identification. Techniques like PCR and capillary electrophoresis utilize labeled probes to amplify and analyze DNA samples, contributing to criminal investigations and paternity testing.
6. Medical Diagnostics: Detecting Pathogens and Genetic Diseases
Labeling techniques are essential for medical diagnostics, allowing for the detection of pathogens, genetic diseases, and cancer. Techniques such as PCR and in situ hybridization use labeled probes to detect specific nucleic acid sequences associated with these conditions.
Challenges and Future Directions
Despite significant advances, challenges remain in nucleic acid labeling:
- Background noise: Non-specific binding of labels can lead to false-positive signals, compromising the accuracy of results.
- Photobleaching: Fluorescent labels can lose their fluorescence over time, limiting the duration of experiments.
- Cost: Some labeling techniques, particularly those involving specialized probes or antibodies, can be expensive.
Future research will likely focus on:
- Developing novel labels: Improved labels with enhanced brightness, photostability, and specificity are needed to enhance the sensitivity and accuracy of labeling techniques.
- Multiplexing: Techniques that allow simultaneous labeling of multiple targets will enable the study of complex biological systems.
- Automation: Automation of labeling procedures will increase throughput and reduce the cost of experiments.
- In vivo imaging: Development of methods to label and image nucleic acids in living organisms will provide unique insights into dynamic cellular processes.
Conclusion
The art of labeling nucleic acids is a powerful tool for exploring the structure and function of these vital molecules. A wide array of techniques, from radioactive isotopes to sophisticated affinity-based methods, allows researchers to visualize, quantify, and manipulate DNA and RNA. The applications span a vast range of biological and medical disciplines, driving advancements in our understanding of life's fundamental processes and contributing to the development of new diagnostic and therapeutic tools. As technology continues to evolve, we can expect even more sophisticated labeling methods to emerge, further enhancing our ability to unravel the intricacies of the nucleic acid world.
Latest Posts
Latest Posts
-
Their Eyes Were Watching God Chapter 11 Summary
Apr 27, 2025
-
What Compositional Technique Is Illustrated By The Following Excerpt
Apr 27, 2025
-
This Is Just To Say Themes
Apr 27, 2025
-
Summary Of Chapter 2 The Pearl
Apr 27, 2025
-
Lewis Dot Structures Report Sheet Answers
Apr 27, 2025
Related Post
Thank you for visiting our website which covers about Art-labeling Activity Structure Of The Nucleic Acids Dna And Rna . We hope the information provided has been useful to you. Feel free to contact us if you have any questions or need further assistance. See you next time and don't miss to bookmark.