Assign A Molecular Geometry To Each Interior Atom In Cytosine
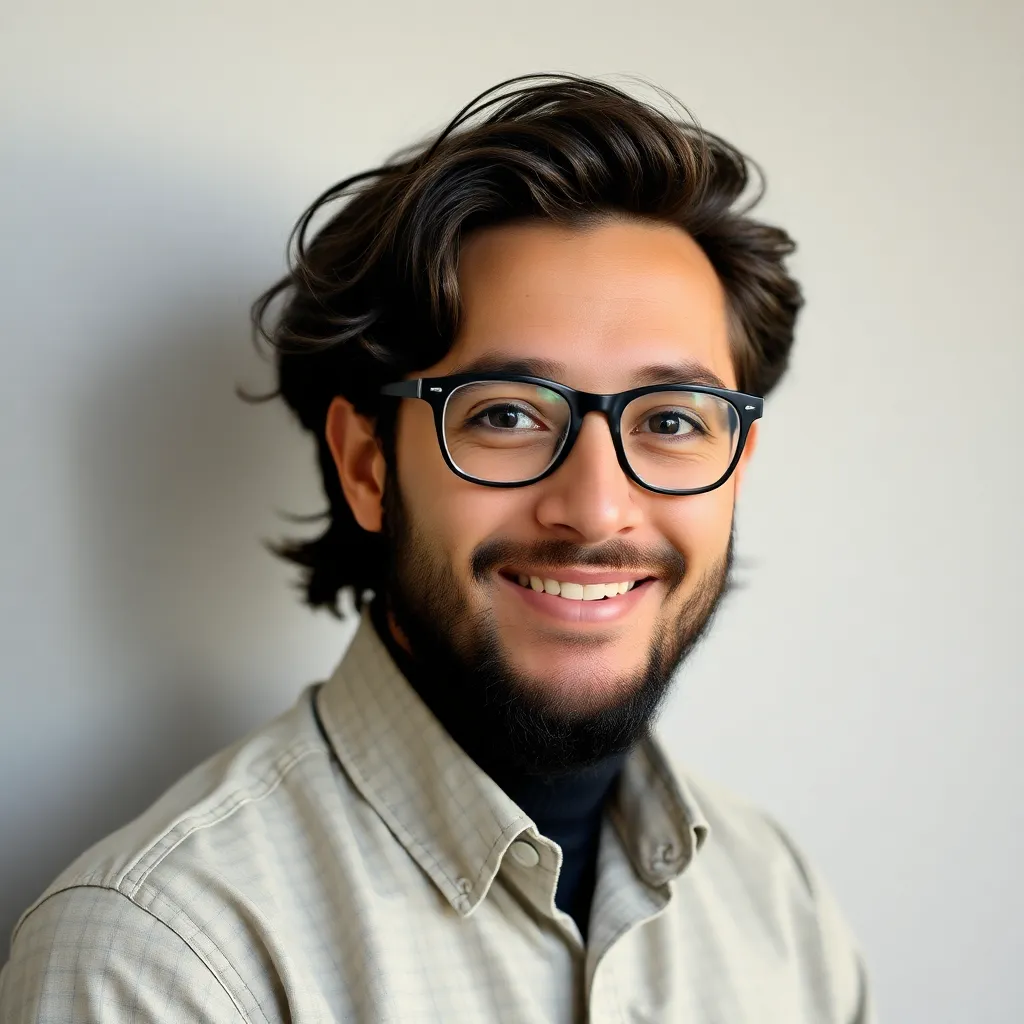
Onlines
May 08, 2025 · 5 min read
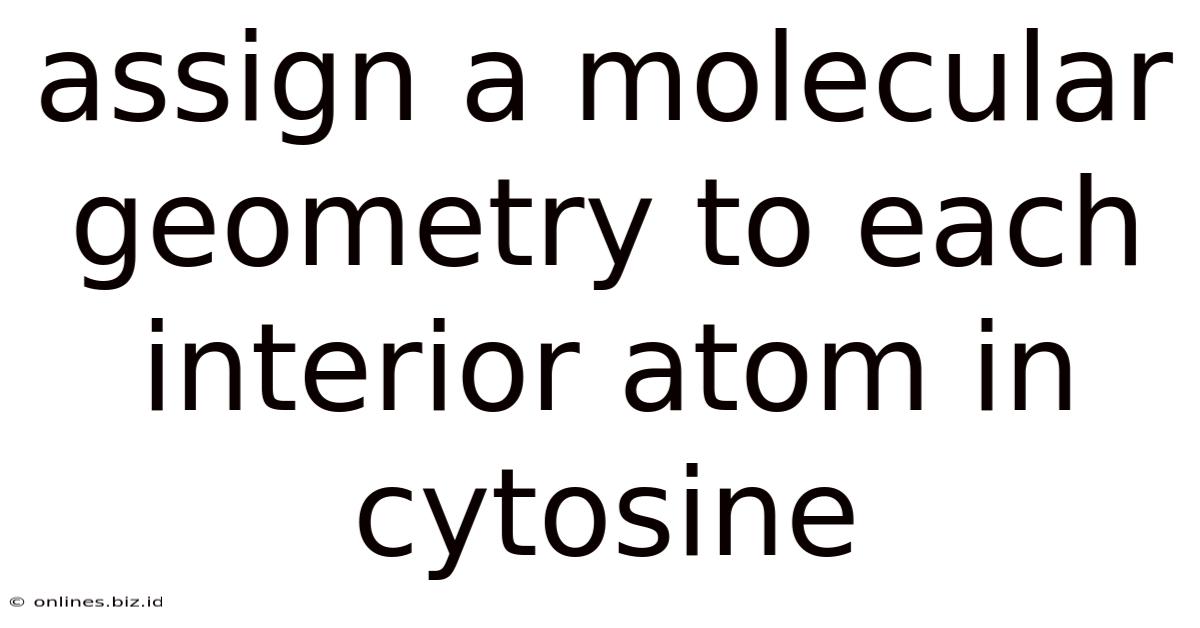
Table of Contents
Assigning Molecular Geometry to Each Interior Atom in Cytosine: A Comprehensive Guide
Cytosine, a fundamental nucleobase in RNA and DNA, possesses a rich and intricate molecular structure. Understanding its geometry is crucial for grasping its role in genetic information storage and transfer, protein synthesis, and numerous biological processes. This article delves into the detailed molecular geometry of cytosine, assigning a specific geometry to each interior atom based on VSEPR theory (Valence Shell Electron Pair Repulsion theory). We'll explore the underlying principles, examine the individual atoms, and discuss the implications of cytosine's geometry for its biological function.
Understanding VSEPR Theory and its Application to Cytosine
VSEPR theory provides a powerful framework for predicting the three-dimensional arrangement of atoms in a molecule. It posits that electron pairs, both bonding and non-bonding (lone pairs), around a central atom repel each other, leading to a geometry that maximizes the distance between them. The number of electron pairs dictates the basic geometry, while the presence of lone pairs influences the final molecular shape.
To apply VSEPR theory to cytosine, we must consider each interior atom individually, identifying the number of sigma bonds and lone pairs associated with it. This will allow us to predict the electron-pair geometry and the resulting molecular geometry. Cytosine's structure contains several different atom types, each with unique bonding environments.
Detailed Geometry Analysis of Cytosine's Interior Atoms
Cytosine's chemical formula is C₄H₅N₃O. Let's analyze each interior atom:
1. Nitrogen Atoms (N1, N3, N4)
-
N1: This nitrogen atom is bonded to two carbon atoms (C2 and C6) and possesses one lone pair of electrons. This gives it a steric number of 3 (2 bonds + 1 lone pair). According to VSEPR, this configuration leads to a trigonal planar electron-pair geometry. The presence of the lone pair slightly distorts the molecular geometry, making the bond angles less than 120 degrees. However, it's still considered essentially trigonal planar.
-
N3: This nitrogen atom forms a double bond with C4 and a single bond with H. It also has one lone pair. Similar to N1, this results in a steric number of 3. Therefore, N3 also exhibits a trigonal planar electron-pair geometry with a slightly distorted molecular geometry due to the lone pair.
-
N4: This nitrogen atom is bonded to two carbon atoms (C5 and C6) and has one lone pair. This gives it a steric number of 3, leading to a trigonal planar electron-pair geometry. Again, the lone pair causes a slight distortion in the bond angles, but the overall geometry remains trigonal planar.
2. Carbon Atoms (C2, C4, C5, C6)
-
C2: This carbon atom is bonded to N1, N3, and one hydrogen atom (H). This means it has three sigma bonds, and zero lone pairs, resulting in a steric number of 3. The geometry is trigonal planar. The bond angles are very close to 120°.
-
C4: This carbon atom forms a double bond with N3 and a single bond with C5. It has no lone pairs. This results in a steric number of 2. The geometry is linear. The atoms bonded to C4 lie essentially in a straight line.
-
C5: This carbon atom forms single bonds with C4 and C6, and also has a single bond with a hydrogen atom. This gives it a steric number of 3, leading to a trigonal planar geometry.
-
C6: This carbon atom is bonded to N1, N4, and C5. It has three sigma bonds and zero lone pairs. Therefore, its steric number is 3, resulting in a trigonal planar geometry.
3. Oxygen Atom (O2)
- O2: The oxygen atom is double bonded to C2. It has two lone pairs of electrons. Its steric number is 2. Therefore, the electron-pair geometry is linear, but the molecular geometry is also essentially linear since the two double bonds are positioned opposite each other.
Implications of Cytosine's Geometry for its Biological Function
The specific geometries of the atoms within cytosine are not merely an academic exercise; they have crucial implications for its biological role.
-
Hydrogen Bonding: The planar structure of cytosine, particularly the arrangement of the nitrogen and oxygen atoms, is critical for its ability to form hydrogen bonds with guanine. These hydrogen bonds are the basis of the complementary base pairing in DNA and RNA, crucial for DNA replication, transcription, and translation. The specific geometry ensures optimal hydrogen bond formation and stability.
-
Stacking Interactions: The planar nature of cytosine also facilitates stacking interactions with adjacent bases within the DNA double helix. These stacking interactions contribute significantly to the stability of the DNA structure. A non-planar structure would disrupt these crucial interactions.
-
Molecular Recognition: The precise spatial arrangement of atoms in cytosine allows for specific recognition by enzymes involved in DNA replication, repair, and other processes. Any alteration in its geometry could impair its interaction with these enzymes.
Advanced Considerations and Conclusion
While VSEPR theory provides a good first-order approximation of molecular geometry, more sophisticated computational methods, such as density functional theory (DFT), can offer a more accurate and nuanced representation of the electron distribution and bond angles in cytosine. These methods account for factors not explicitly considered in VSEPR, such as electron correlation and orbital hybridization.
In conclusion, a detailed analysis of cytosine's structure using VSEPR theory reveals a molecule with primarily trigonal planar and linear geometries around its interior atoms. These geometries are not arbitrary but are fundamental to cytosine's ability to participate in crucial biological functions such as hydrogen bonding, base stacking, and molecular recognition. Understanding the relationship between molecular structure and function in cytosine enhances our understanding of the intricacies of molecular biology and the processes of life itself. Further exploration using advanced computational methods can provide a more refined understanding of the subtle nuances in the geometry and its implications for biological activity. The precise arrangement of atoms in cytosine is a testament to the elegance and efficiency of biological design.
Latest Posts
Latest Posts
-
What Is Johns Response To Her Prodding
May 08, 2025
-
The Department Of The Navy Has What Principal Components
May 08, 2025
-
What Type Of Market Is Illustrated By This Pie Chart
May 08, 2025
-
A Collection Of Nickels And Dimes Is Worth 9 45
May 08, 2025
-
This Paragraph Supports The Idea That Micaela
May 08, 2025
Related Post
Thank you for visiting our website which covers about Assign A Molecular Geometry To Each Interior Atom In Cytosine . We hope the information provided has been useful to you. Feel free to contact us if you have any questions or need further assistance. See you next time and don't miss to bookmark.