Assign An Electron Geometry To Each Interior Atom In Cytosine
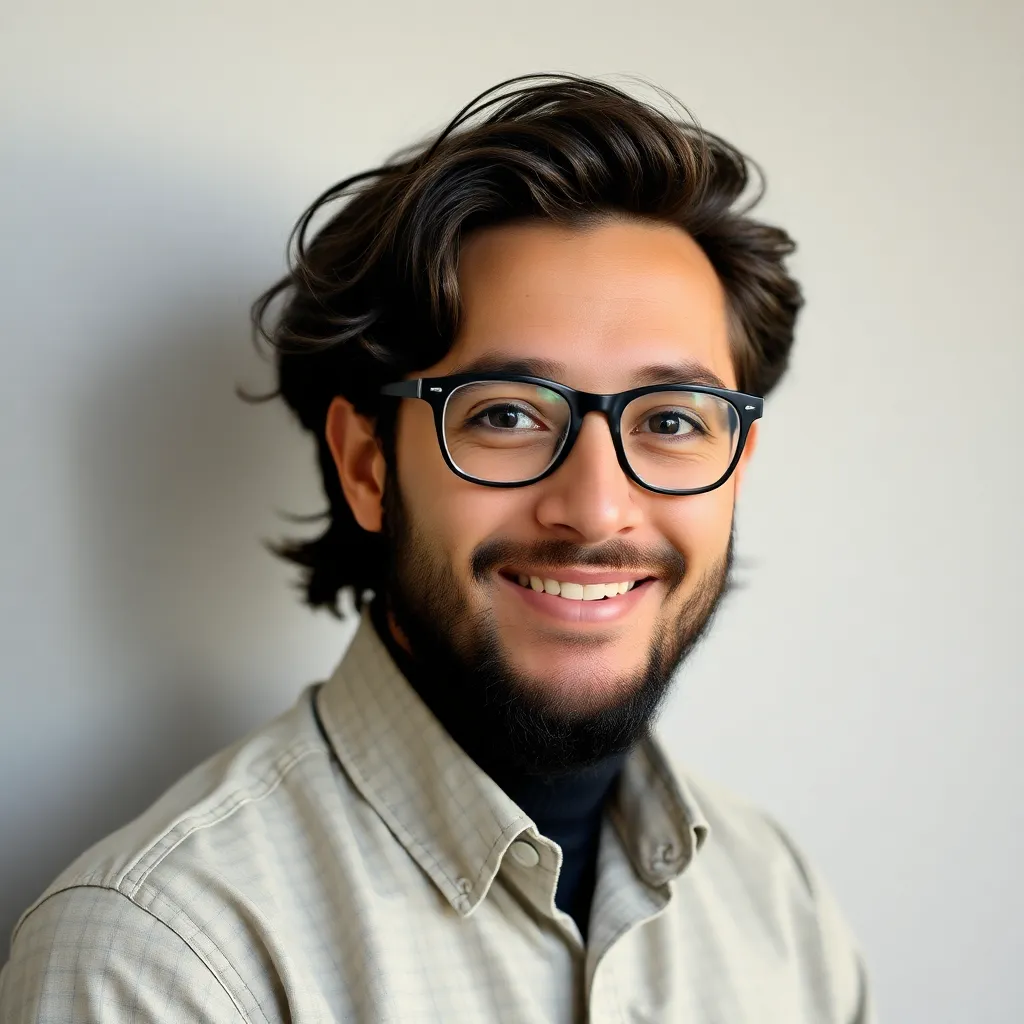
Onlines
May 12, 2025 · 6 min read
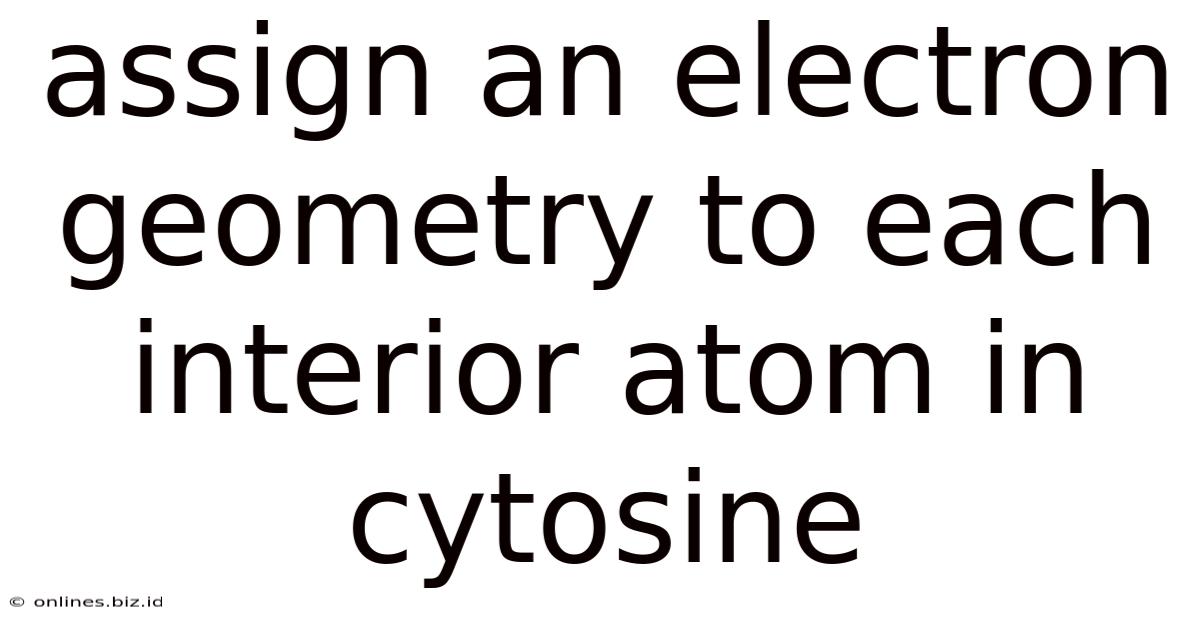
Table of Contents
Assigning Electron Geometry to Each Interior Atom in Cytosine: A Comprehensive Guide
Cytosine, a fundamental nucleobase in DNA and RNA, presents a fascinating case study for understanding molecular geometry. Its relatively small size belies a rich complexity of bonding and electron distribution, making it an ideal molecule for exploring concepts like VSEPR theory and electron geometry. This article will meticulously detail the electron geometry around each interior atom within cytosine, providing a clear, step-by-step explanation accessible to both beginners and advanced learners. We'll delve into the underlying principles, explore the nuances of bonding, and illuminate the connection between electron geometry and molecular shape.
Understanding VSEPR Theory
Before diving into the specifics of cytosine, it's crucial to establish a firm understanding of the Valence Shell Electron Pair Repulsion (VSEPR) theory. VSEPR theory is a cornerstone of molecular geometry prediction. It postulates that the electron pairs surrounding a central atom arrange themselves to minimize repulsion, thus dictating the molecule's three-dimensional shape. This arrangement is primarily determined by the number of bonding and non-bonding (lone) electron pairs.
Key VSEPR Concepts:
- Electron Domains: These encompass both bonding pairs (shared electrons between atoms) and lone pairs (unshared electrons associated with an atom).
- Minimizing Repulsion: The electron domains arrange themselves as far apart as possible to minimize electrostatic repulsion.
- Geometry Prediction: The number of electron domains dictates the overall electron geometry, while the number of lone pairs influences the molecular geometry (the actual arrangement of atoms).
Cytosine's Structure: A Foundation for Geometry Analysis
Cytosine (C₄H₅N₃O) possesses a six-membered heterocyclic ring incorporating nitrogen and oxygen atoms. Its structure is characterized by several key bonding features that are critical to determining the electron geometry around each atom:
- Nitrogen Atoms: Nitrogen atoms contribute significantly to the bonding network and often exhibit lone pairs.
- Oxygen Atom: The oxygen atom displays two lone pairs in addition to its bonding electrons.
- Carbon Atoms: Carbon atoms form the backbone of the ring structure, primarily exhibiting sigma and pi bonds.
- Hydrogen Atoms: These atoms primarily contribute to the overall molecular structure via single bonds.
Detailed Electron Geometry Analysis of Cytosine's Interior Atoms
Let's systematically analyze the electron geometry around each interior atom (carbon, nitrogen, and oxygen) in cytosine. We'll follow a consistent approach: identifying the number of electron domains, determining the electron geometry based on the VSEPR theory, and then noting any deviations caused by lone pairs.
Nitrogen Atoms (N1, N3, and N4):
-
N1: This nitrogen atom is sp2 hybridized. It forms three sigma bonds (two with carbon atoms and one with a hydrogen atom) and possesses one lone pair of electrons. Therefore, it has four electron domains. According to VSEPR, this leads to a tetrahedral electron geometry. However, the presence of a lone pair modifies the molecular geometry to trigonal planar.
-
N3: Similar to N1, N3 is also sp2 hybridized. It forms three sigma bonds (two with carbon atoms and one with a hydrogen atom) and has one lone pair of electrons. This results in four electron domains, leading to a tetrahedral electron geometry. The molecular geometry is also trigonal planar due to the presence of the lone pair.
-
N4: This nitrogen atom has different bonding characteristics. It forms two sigma bonds (one with a carbon atom and one with a hydrogen atom) and has two lone pairs of electrons. This means there are four electron domains, again leading to a tetrahedral electron geometry. The molecular geometry, however, is bent due to the presence of two lone pairs.
Oxygen Atom (O2):
- O2: The oxygen atom (O2) is sp2 hybridized. It forms two sigma bonds (one with a carbon and one with a hydrogen) and it possesses two lone pairs. Therefore it has four electron domains giving it a tetrahedral electron geometry. The presence of two lone pairs results in a bent molecular geometry.
Carbon Atoms (C2, C4, C5, C6):
- C2, C4, C5, C6: Each of these carbon atoms is sp2 hybridized. They each form three sigma bonds (either with other carbons, nitrogens or one oxygen) and have one pi bond. This means each carbon atom has three sigma bonds and one pi bond, resulting in three electron domains. Therefore, these carbon atoms exhibit a trigonal planar electron geometry and a trigonal planar molecular geometry.
Implications of Electron Geometry
The electron geometry around each atom within cytosine directly influences its overall three-dimensional shape and reactivity. The electron distribution dictates the orientation of the various functional groups and plays a key role in its interactions with other molecules, such as during base pairing in DNA.
Key Implications:
- Base Pairing: The specific arrangement of atoms and lone pairs in cytosine is crucial for its ability to form hydrogen bonds with guanine, a fundamental aspect of DNA structure and function.
- Reactivity: The electron density distribution and the presence of lone pairs influence the sites where cytosine can undergo chemical reactions.
- Molecular Recognition: The three-dimensional shape of cytosine, determined by its electron geometry, is essential for its recognition by enzymes and other proteins involved in DNA replication, repair, and transcription.
Advanced Considerations: Hybridization and Molecular Orbitals
While VSEPR provides an excellent framework for understanding the general electron geometry, a more in-depth understanding necessitates considering atomic hybridization and molecular orbitals.
- Hybridization: The concept of hybridization describes how atomic orbitals combine to form hybrid orbitals with different shapes and energies. In cytosine, the sp2 hybridization of carbon and nitrogen atoms is crucial in forming the planar structure of the ring.
- Molecular Orbitals: Molecular orbital theory provides a more sophisticated picture of electron distribution within the molecule. It describes how atomic orbitals combine to form molecular orbitals that extend over the entire molecule, providing a more accurate description of bonding and electron density.
Conclusion: A Holistic Perspective of Cytosine's Geometry
Assigning electron geometry to each interior atom in cytosine provides a comprehensive understanding of its structure and properties. By applying VSEPR theory and considering the nuances of hybridization and molecular orbitals, we gain a detailed insight into the electron distribution and its implications for reactivity, base pairing, and molecular recognition. This analysis highlights the interconnectedness of structure and function at the molecular level and underscores the significance of understanding electron geometry in biological systems. The information presented in this article can be useful for students of chemistry, biochemistry, and molecular biology, providing a solid foundation for further exploration of the intricate world of molecular structure and function. This approach serves as a powerful tool for predicting and explaining the behavior of more complex molecules. The deep dive into the structure of cytosine helps in understanding the fundamental principles of molecular geometry and its significance in the field of life sciences. Further exploration could involve investigating how these principles apply to other nucleobases and the broader field of organic chemistry.
Latest Posts
Related Post
Thank you for visiting our website which covers about Assign An Electron Geometry To Each Interior Atom In Cytosine . We hope the information provided has been useful to you. Feel free to contact us if you have any questions or need further assistance. See you next time and don't miss to bookmark.