Classify The Metabolic Poisons As Electron Transport Inhibitors
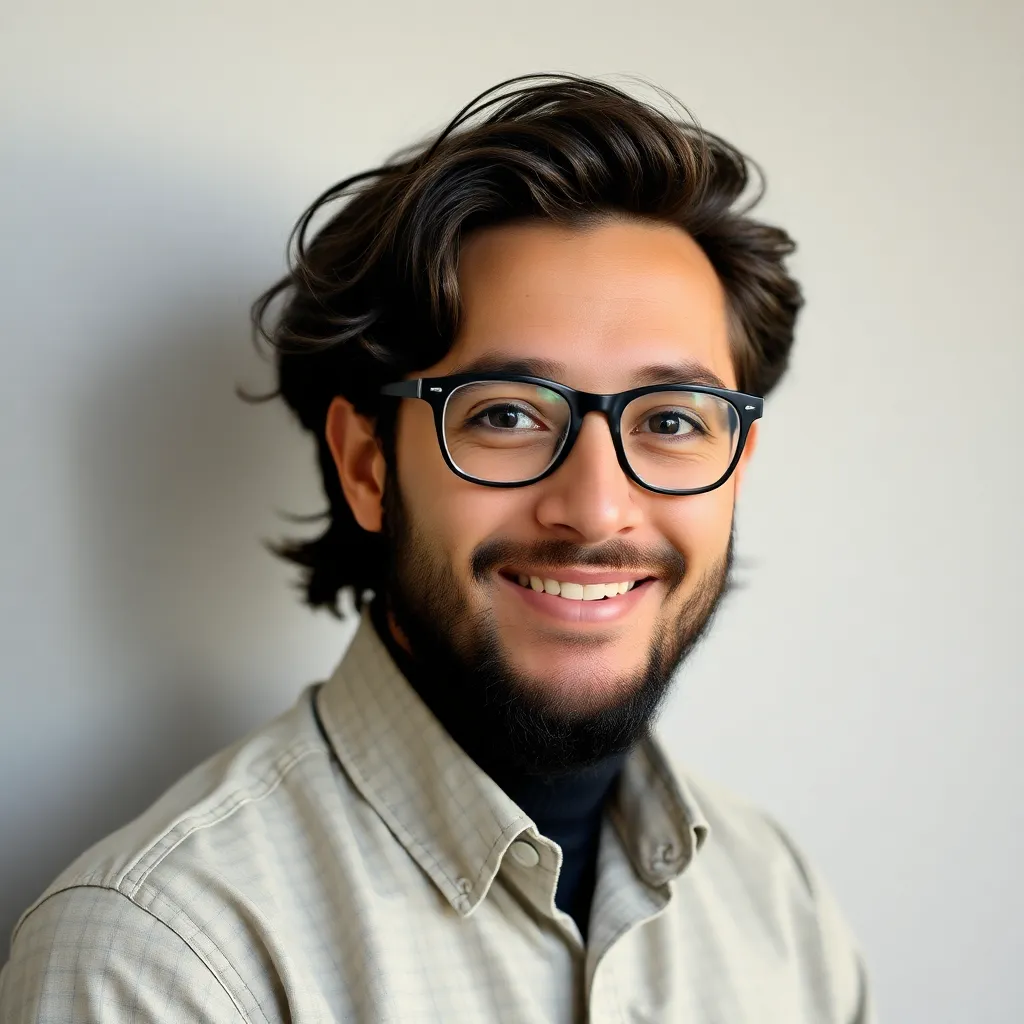
Onlines
May 09, 2025 · 6 min read
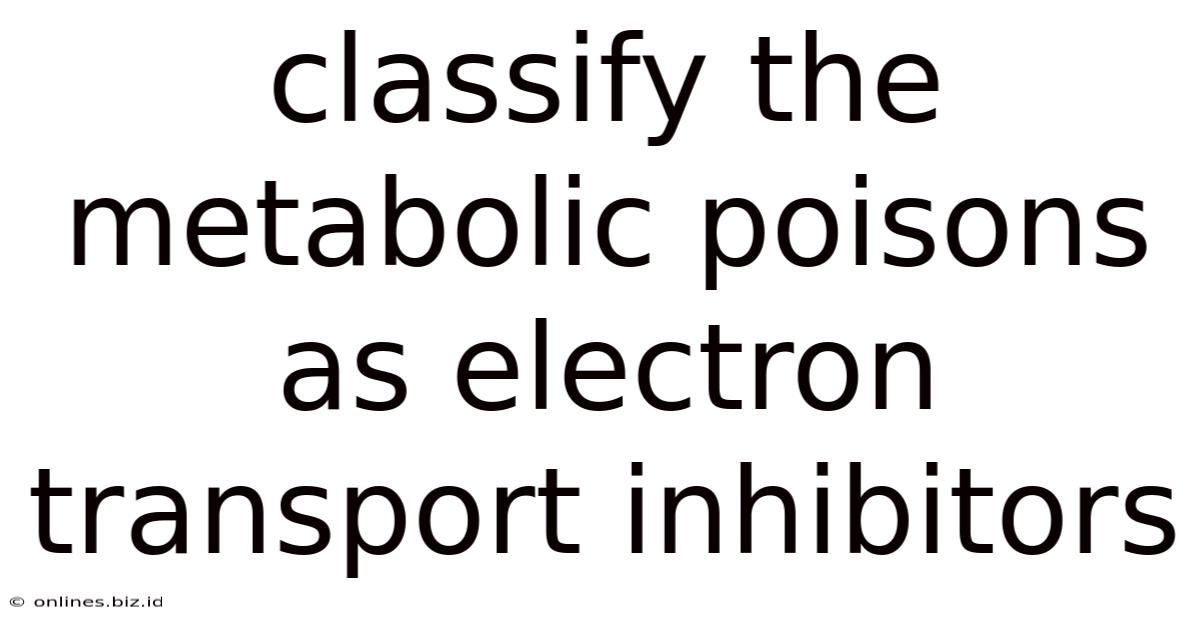
Table of Contents
Classifying Metabolic Poisons as Electron Transport Inhibitors
Electron transport chain (ETC) inhibitors are a class of metabolic poisons that interfere with the process of oxidative phosphorylation, a crucial step in cellular respiration responsible for generating the majority of ATP (adenosine triphosphate), the cell's primary energy currency. These poisons disrupt the flow of electrons along the ETC, ultimately halting ATP production and leading to cellular damage and, potentially, death. Understanding their classification is vital in toxicology, pharmacology, and the study of mitochondrial function. This article delves deep into the classification of metabolic poisons as ETC inhibitors, exploring their mechanisms of action and diverse effects on cellular metabolism.
Mechanisms of Electron Transport Chain Inhibition
ETC inhibitors achieve their toxic effects by targeting specific components of the electron transport chain within the inner mitochondrial membrane. They can be broadly classified based on their site of action:
Complex I Inhibitors:
These poisons block the transfer of electrons from NADH to ubiquinone (coenzyme Q) at Complex I (NADH dehydrogenase). This blockage prevents the pumping of protons across the inner mitochondrial membrane, disrupting the proton gradient essential for ATP synthesis. Examples include:
-
Rotenone: A naturally occurring insecticide derived from plants, rotenone is a potent Complex I inhibitor. Its mechanism involves binding to a specific site within Complex I, preventing electron transfer and leading to the accumulation of NADH. This can cause significant cellular damage and is associated with Parkinson's disease pathogenesis in some research.
-
Amytal (Amobarbital): A barbiturate drug, amytal also inhibits Complex I. While used medically as a sedative and anesthetic, its effects on the ETC highlight its potential for toxicity at higher doses or in individuals with impaired metabolic function.
-
Piericidin A: A naturally occurring antibiotic, piericidin A is another strong Complex I inhibitor. Its mechanism is similar to rotenone, directly blocking electron transfer.
Complex II Inhibitors:
Though less common than Complex I inhibitors, some poisons target Complex II (succinate dehydrogenase). Complex II is unique in that it's part of both the citric acid cycle and the ETC. Inhibition at this site impacts both energy production and intermediary metabolism. While specific examples of direct Complex II inhibitors as metabolic poisons are less prominent in the literature, the implications of interfering with this complex are significant and warrant consideration. Indirect effects on Complex II can occur through the disruption of the citric acid cycle.
Complex III Inhibitors:
Complex III (cytochrome bc1 complex) is a critical juncture in the ETC. Several poisons effectively target this complex:
-
Antimycin A: A naturally occurring antibiotic, antimycin A is a well-known and potent Complex III inhibitor. It binds to the ubiquinol-binding site of Complex III, preventing the transfer of electrons to cytochrome c. This blockage leads to the accumulation of reduced ubiquinol and a significant decrease in ATP production.
-
Myxothiazol: Similar to antimycin A, myxothiazol is a naturally occurring antibiotic that inhibits Complex III. It binds to a different site within the complex, offering insights into the multiple vulnerabilities within Complex III.
Complex IV Inhibitors:
Complex IV (cytochrome c oxidase) catalyzes the final electron transfer step in the ETC, reducing oxygen to water. Several substances inhibit this crucial step:
-
Cyanide (CN⁻): Cyanide is a highly toxic and rapidly acting poison that directly binds to the heme a3 in Complex IV, preventing the reduction of oxygen. This leads to complete shutdown of the ETC and rapid cellular death. Its potency and rapid action make it a significant toxicological concern.
-
Azide (N₃⁻): Similar to cyanide, azide also binds to the heme a3 in Complex IV, inhibiting oxygen reduction. While less potent than cyanide, azide is still a significant respiratory inhibitor.
-
Carbon Monoxide (CO): Carbon monoxide is a colorless, odorless gas that competitively binds to the heme a3 in Complex IV, preventing oxygen binding and electron transfer. Its toxicity is widely known, particularly concerning its effects on the respiratory system.
-
Hydrogen Sulfide (H₂S): Hydrogen sulfide, a toxic gas, also inhibits Complex IV by binding to the heme iron of cytochrome c oxidase, impeding electron transport and oxygen reduction.
ATP Synthase Inhibitors:
While not directly part of the electron transport chain, ATP synthase is the enzyme responsible for ATP synthesis using the proton gradient established by the ETC. Inhibiting ATP synthase effectively stops ATP production even if the electron transport chain is functioning.
- Oligomycin: This antibiotic is a potent inhibitor of ATP synthase, preventing the synthesis of ATP from ADP and inorganic phosphate. Its effects highlight the critical role of ATP synthase in the overall process of energy production.
Consequences of Electron Transport Chain Inhibition
The consequences of ETC inhibition are far-reaching and depend on the severity and duration of the inhibition. Key effects include:
-
Reduced ATP Production: This is the most immediate and critical consequence, leading to a cellular energy crisis. Cells rely heavily on ATP for virtually all their functions, including active transport, protein synthesis, and muscle contraction.
-
Increased Reactive Oxygen Species (ROS): When electron flow through the ETC is blocked, electrons can "leak" from the chain and react with oxygen, forming ROS such as superoxide radicals. These ROS are highly damaging to cellular components, including lipids, proteins, and DNA, leading to oxidative stress and cell damage.
-
Cellular Necrosis or Apoptosis: Depending on the severity and duration of the inhibition, cells may undergo necrosis (uncontrolled cell death) or apoptosis (programmed cell death). The type of cell death is influenced by various factors, including the concentration of the poison, the cell type, and the presence of protective mechanisms.
-
Organ System Dysfunction: The widespread effects of ETC inhibition can lead to dysfunction in multiple organ systems, ultimately leading to death. The specific organ systems affected depend on the specific poison and the route of exposure.
Clinical Significance and Therapeutic Implications
Understanding the classification and mechanisms of ETC inhibitors is crucial in various fields:
-
Toxicology: Identifying and treating poisoning caused by ETC inhibitors requires a thorough understanding of their mechanisms of action and the resulting physiological effects. Specific antidotes exist for some poisons, such as cyanide (e.g., sodium thiosulfate).
-
Pharmacology: Some ETC inhibitors are used therapeutically, although their use is carefully controlled due to their potential toxicity. For example, some antibiotics target bacterial ETC components, selectively inhibiting bacterial growth while minimizing harm to the host.
-
Disease Research: ETC dysfunction is implicated in various diseases, including Parkinson's disease, Alzheimer's disease, and cancer. Studying the mechanisms of ETC inhibition can provide insights into disease pathogenesis and potential therapeutic targets.
Conclusion
The classification of metabolic poisons as electron transport chain inhibitors offers a structured approach to understanding the diverse mechanisms by which these poisons disrupt cellular respiration. By targeting various components of the ETC, from Complex I to Complex IV and even ATP synthase, these poisons profoundly impact cellular energy production and homeostasis. The consequences, ranging from reduced ATP levels to increased ROS production and cellular death, highlight the critical role of the ETC in maintaining life. Further research into the specific mechanisms of action of these inhibitors, along with the development of effective antidotes and therapeutic strategies, remains a crucial area of ongoing investigation. Understanding the intricacies of ETC inhibition is essential not only for mitigating the toxic effects of these compounds but also for advancing our knowledge of cellular metabolism and disease pathogenesis.
Latest Posts
Latest Posts
-
A Differentiation Strategy Works Best When
May 11, 2025
-
Which Statement Is An Evaluation Of Mortimers Writing Style
May 11, 2025
-
Air Carriers Were Historically Looked Upon As
May 11, 2025
-
Economic Systems And Decision Making Guided Reading Activity
May 11, 2025
-
The Significance Of The Frontier In American History Summary
May 11, 2025
Related Post
Thank you for visiting our website which covers about Classify The Metabolic Poisons As Electron Transport Inhibitors . We hope the information provided has been useful to you. Feel free to contact us if you have any questions or need further assistance. See you next time and don't miss to bookmark.