Correctly Identify The Following Parts Of Transmembrane Proteins
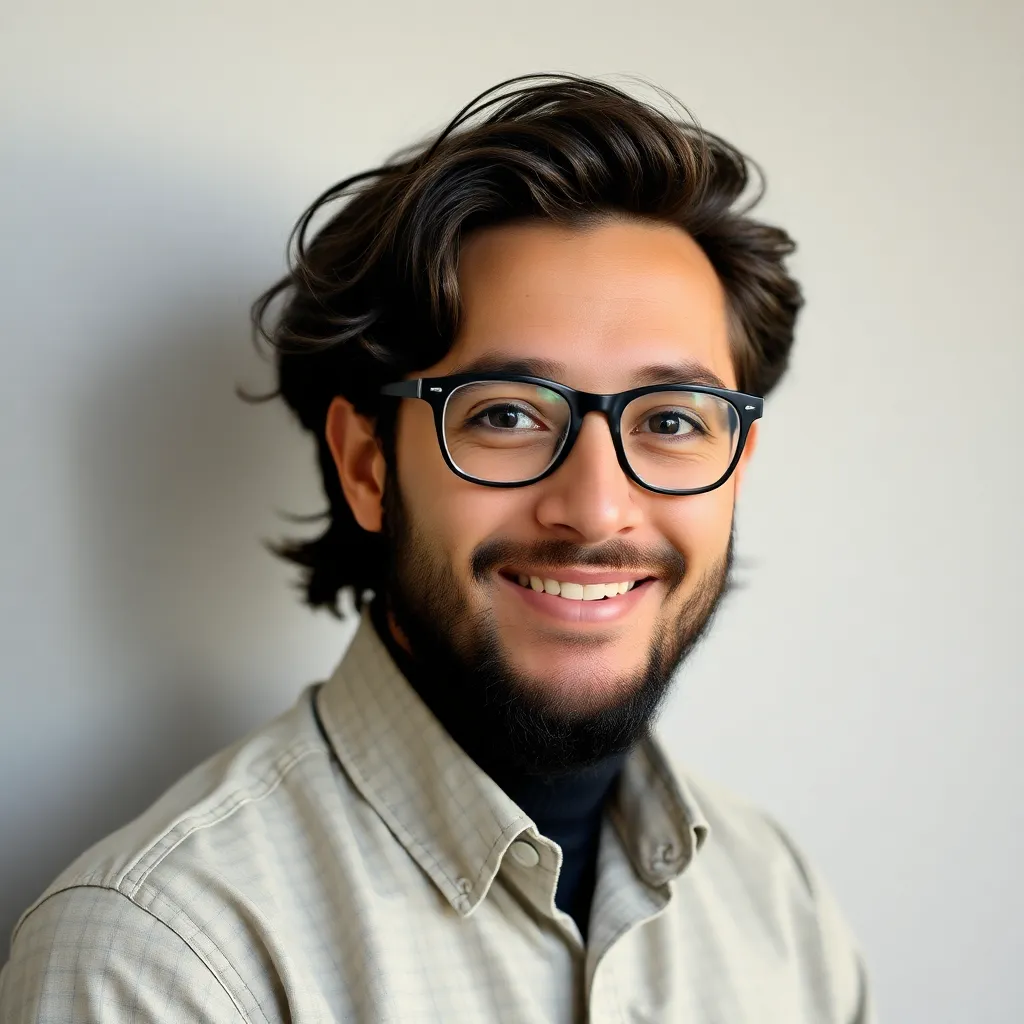
Onlines
May 08, 2025 · 6 min read
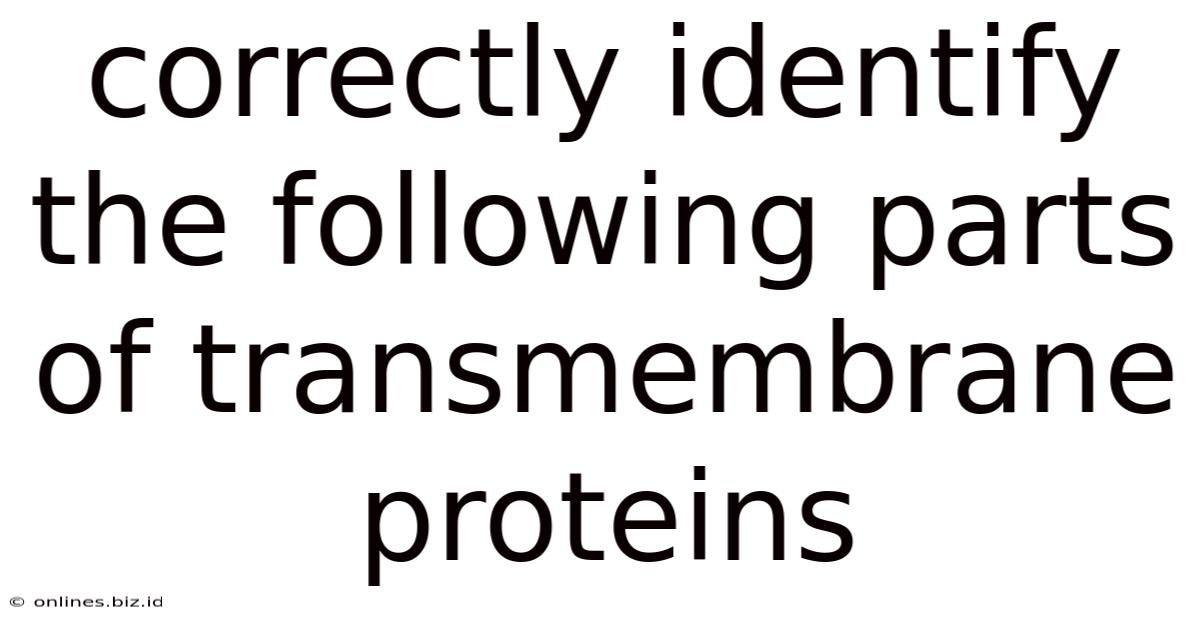
Table of Contents
Correctly Identifying the Parts of Transmembrane Proteins
Transmembrane proteins (TMPs) are vital components of cell membranes, acting as gatekeepers controlling the passage of molecules and ions, facilitating cell signaling, and mediating various cellular processes. Understanding their structure is key to grasping their function. This comprehensive guide will delve into the intricacies of TMP structure, helping you correctly identify their constituent parts.
The Core Components of Transmembrane Proteins
TMPs are defined by their ability to span the hydrophobic lipid bilayer of the cell membrane. This necessitates specific structural features that allow them to interact favorably with both the hydrophilic aqueous environments and the hydrophobic lipid core. Let's break down the key components:
1. Transmembrane Domains (TMDs): The Lipid-Loving Heart
The transmembrane domains (TMDs) are the most defining characteristic of TMPs. These are stretches of predominantly hydrophobic amino acid residues that directly interact with the fatty acyl chains of the lipid bilayer. These residues, such as leucine, isoleucine, valine, phenylalanine, and tryptophan, are crucial for anchoring the protein within the membrane. The TMDs often adopt an alpha-helical or beta-barrel secondary structure, maximizing hydrophobic interactions and minimizing contact with water.
-
Alpha-helices: These are the most common conformation for TMDs. The helical structure buries the hydrophobic side chains inward, away from the aqueous environment, while the backbone hydrogen bonds stabilize the structure. The length of an alpha-helical TMD usually corresponds to the thickness of the lipid bilayer, typically around 20-30 amino acids.
-
Beta-barrels: Found primarily in bacterial outer membranes and some eukaryotic organelles, beta-barrels form a cylindrical structure composed of multiple beta-strands. The hydrophobic side chains are oriented outward towards the lipid bilayer, while the hydrophilic residues line the barrel's interior, creating a pore through the membrane.
2. Extracellular and Intracellular Domains: The Water-Loving Extremes
The portions of the TMP that extend beyond the lipid bilayer are known as the extracellular domain (ECD) and the intracellular domain (ICD). These domains are predominantly hydrophilic, interacting with the aqueous environments on either side of the membrane. Their sequences are rich in charged and polar amino acids, enabling them to form hydrogen bonds with water molecules.
-
Extracellular Domain (ECD): This region often participates in ligand binding, cell-cell adhesion, or enzymatic activity. It can be heavily glycosylated, adding further complexity and functionality. Glycosylation can affect protein folding, stability, and interactions with other molecules.
-
Intracellular Domain (ICD): This domain interacts with intracellular signaling molecules, cytoskeletal components, or other intracellular proteins. Phosphorylation of specific amino acid residues within the ICD often regulates protein activity and interactions.
3. Connecting Regions: Linking the Domains
The ECD and ICD are connected to the TMDs by loop regions, sometimes referred to as connecting regions or linker regions. These are sequences of amino acids that are less ordered and highly flexible compared to the α-helices or β-sheets of the TMDs. They can be short or lengthy, and their length and amino acid composition dictate their flexibility and influence how the transmembrane domains are oriented relative to each other and the membrane. The loops themselves can also participate in interactions and signal transduction, adding a layer of complexity to transmembrane protein function. The nature of these loops, whether they are hydrophilic or contain hydrophobic stretches, can impact how the protein interacts with the membrane and its surrounding environment.
4. Signal Sequences: Directing Protein Insertion
The proper insertion of TMPs into the cell membrane is guided by signal sequences, typically located at the N-terminus. These sequences are short stretches of amino acids that direct the ribosome-nascent chain complex to the endoplasmic reticulum (ER) membrane. Once the protein is synthesized, the signal sequence is cleaved off by signal peptidases. The presence and location of these signal sequences are crucial for the correct targeting and membrane insertion of the TMP. Specific signal sequences dictate where in the cell the protein will reside, for instance, leading to specific cellular compartments, like the mitochondria or chloroplasts.
5. Post-translational Modifications: Fine-tuning Function
TMPs often undergo various post-translational modifications (PTMs) that fine-tune their function. These modifications include glycosylation (addition of sugar moieties), phosphorylation (addition of phosphate groups), lipidation (addition of lipid molecules), and ubiquitination (addition of ubiquitin molecules). These PTMs can affect protein stability, activity, localization, and interactions with other molecules. Identifying these PTMs is crucial for a complete understanding of TMP function. For example, glycosylation in the extracellular domain often plays a crucial role in cell recognition and signaling, while phosphorylation in the intracellular domain can regulate protein interactions and enzyme activity.
Identifying the Parts in Practice: Methods and Considerations
Identifying the specific components of a TMP requires a multi-faceted approach combining bioinformatics and experimental techniques.
1. Bioinformatics: Predicting Structure from Sequence
The amino acid sequence of a TMP provides valuable clues about its structure. Bioinformatics tools utilize algorithms that predict the location of TMDs based on the hydrophobicity of amino acid residues. Software programs like TMHMM, HMMTOP, and Phobius analyze the sequence and provide probability scores for transmembrane segments. These predictions, however, need experimental validation. While highly accurate, bioinformatics methods are only a starting point.
2. Experimental Techniques: Confirming and Characterizing Structure
Experimental validation is crucial for confirming bioinformatics predictions. Techniques like:
-
X-ray crystallography: This high-resolution technique provides detailed three-dimensional structures of proteins, revealing the arrangement of TMDs, ECDs, and ICDs.
-
Nuclear magnetic resonance (NMR) spectroscopy: This technique is also capable of determining protein structures, particularly for smaller or more flexible proteins.
-
Cryo-electron microscopy (cryo-EM): This emerging technique is particularly useful for studying large, membrane-bound protein complexes.
-
Circular dichroism (CD) spectroscopy: This technique provides information on the secondary structure content of the protein, such as the proportion of α-helices and β-sheets.
-
Site-directed mutagenesis: This technique allows researchers to change specific amino acid residues and study the effects on protein function and structure. It can be used to confirm the roles of different domains and residues within the protein.
These methods, when used in combination with bioinformatics tools, can provide a comprehensive understanding of TMP structure. However, each technique has its limitations and must be interpreted in the context of other results.
Challenges and Future Directions
While significant progress has been made in understanding TMP structure, challenges remain. Predicting the precise conformation of loop regions, particularly those connecting the TMDs and the extra- and intracellular domains remains difficult. Also, analyzing the dynamic interactions of TMPs within the complex cellular environment continues to be a challenge. The use of advanced imaging techniques and computational simulations is expanding our capabilities to investigate these intricate details. As technology advances, we can expect even more precise and comprehensive structural and functional descriptions of transmembrane proteins. The development of new bioinformatics tools and enhanced experimental techniques will further improve our capacity to reveal the complexities of these vital cellular components. The ongoing research into TMP structure and function holds immense potential for advancing our understanding of various biological processes and developing novel therapeutic strategies.
This deep dive into the structure and identification of transmembrane proteins provides a strong foundation for understanding their critical roles in cell biology. By integrating bioinformatics prediction with experimental validation, researchers can effectively decipher the intricate arrangement of transmembrane domains, extra- and intracellular regions, and their diverse post-translational modifications. This knowledge empowers us to further probe the complexities of cellular functions and to explore the potential for therapeutic applications.
Latest Posts
Latest Posts
-
According To Psychoanalytic Therapy Human Beings Are
May 08, 2025
-
What Is An Example Of Applying Cadence Based Synchronization In Safe
May 08, 2025
-
Photosynthesis Is A Step In The Global Nitrogen Cycle
May 08, 2025
-
A Criminals Modus Operandi Involves The Details Of
May 08, 2025
-
How Does The Author Portray Scotty In This Excerpt
May 08, 2025
Related Post
Thank you for visiting our website which covers about Correctly Identify The Following Parts Of Transmembrane Proteins . We hope the information provided has been useful to you. Feel free to contact us if you have any questions or need further assistance. See you next time and don't miss to bookmark.