Design A Synthesis Of 4-hexanolide From 4-oxohexanal
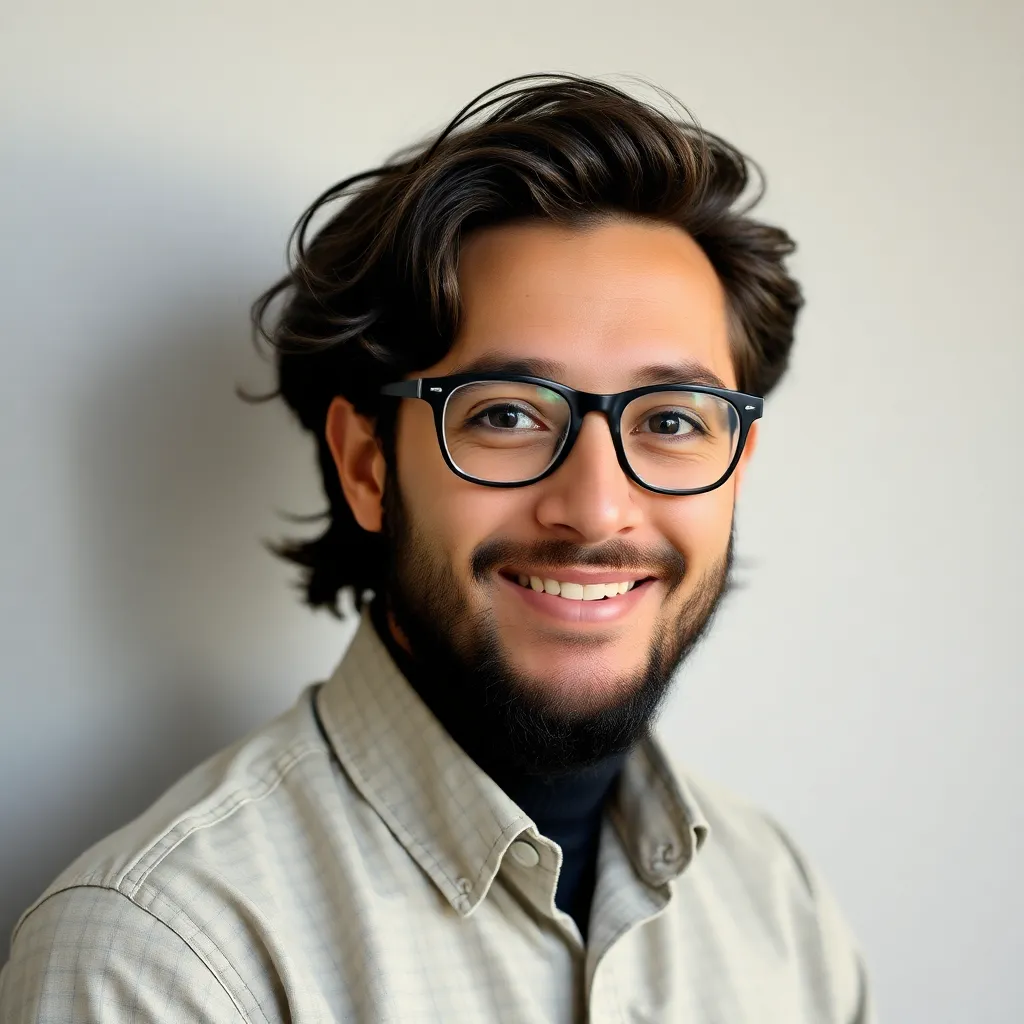
Onlines
May 09, 2025 · 5 min read
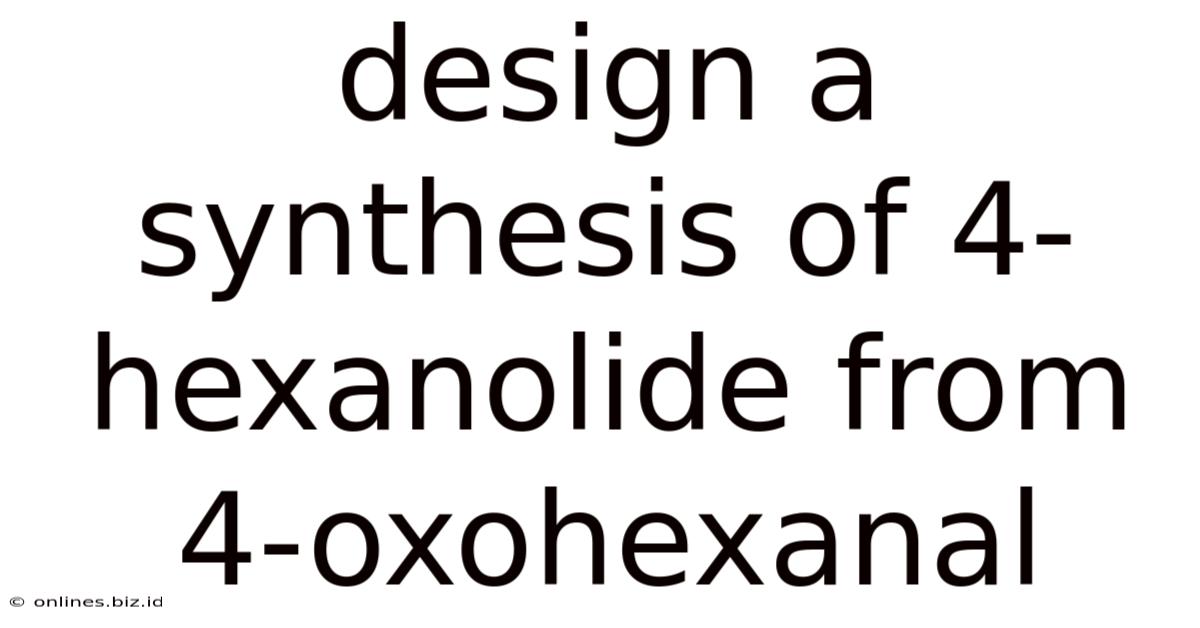
Table of Contents
Designing a Synthesis of 4-Hexanolide from 4-Oxohexanal
The synthesis of 4-hexanolide from 4-oxohexanal presents a fascinating challenge in organic chemistry, requiring a strategic approach to achieve the desired lactonization. This article will delve into the design of a viable synthetic route, exploring different strategies, considering reaction mechanisms, and optimizing for yield and efficiency. We will also discuss potential challenges and alternative approaches.
Understanding the Transformation
The conversion of 4-oxohexanal to 4-hexanolide requires the formation of a five-membered cyclic ester (lactone). This involves a crucial cyclization step, where the aldehyde functional group reacts with the hydroxyl group generated from the ketone. A direct reaction between the aldehyde and ketone is unlikely due to the lack of a suitable leaving group on the carbonyl carbon of the ketone. Therefore, a multi-step approach is necessary.
Proposed Synthetic Route: A Step-by-Step Approach
This synthesis proposes a three-step strategy:
Step 1: Reduction of the Ketone to a Hydroxyl Group
The first step focuses on selectively reducing the ketone group in 4-oxohexanal to a secondary alcohol. Several reducing agents can achieve this transformation, each with its own advantages and disadvantages.
-
Sodium borohydride (NaBH₄): This is a mild reducing agent, selective for ketones in the presence of aldehydes. It reduces the ketone to the corresponding secondary alcohol without affecting the aldehyde. The reaction is typically carried out in a protic solvent like methanol or ethanol.
-
Lithium aluminum hydride (LiAlH₄): While a powerful reducing agent capable of reducing both aldehydes and ketones, LiAlH₄ is less suitable here due to the potential for over-reduction of the aldehyde. Careful control of reaction conditions is crucial to avoid this.
-
Enzymatic reduction: Biocatalytic approaches utilizing enzymes like ketoreductases offer high selectivity and milder reaction conditions, potentially leading to improved yields and reduced waste.
Reaction Scheme (Step 1):
O OH
|| |
CH₃CH₂CH₂C-CH₂CHO --[Reducing Agent] --> CH₃CH₂CH₂CH-CH₂CHO
Step 2: Protection of the Aldehyde Group
The aldehyde group is highly reactive and needs protection to prevent unwanted side reactions during the subsequent cyclization step. Common protecting groups for aldehydes include acetals and cyclic acetals.
-
Acetal protection: Reaction with a diol (e.g., ethylene glycol) in the presence of an acid catalyst forms a cyclic acetal, protecting the aldehyde while leaving the hydroxyl group free for reaction.
-
Other protecting groups: Other protecting groups such as 1,2-ethanedithiol or dimethylacetal are also viable options. The selection depends on the ease of removal and compatibility with subsequent reactions.
Reaction Scheme (Step 2):
OH OH
| |
CH₃CH₂CH₂CH-CH₂CHO --[Protecting Group] --> CH₃CH₂CH₂CH-CH(OR)₂
(where (OR)₂ represents the protecting group derived from the diol)
Step 3: Lactonization and Deprotection
This crucial step involves the formation of the lactone ring. The protected 4-hydroxyhexanal derivative undergoes intramolecular esterification to form the cyclic ester. This often requires acidic conditions to promote the reaction. Subsequently, the protecting group is removed under acidic conditions to unveil the desired 4-hexanolide.
Several methods can be used for lactonization, including:
-
Acid-catalyzed cyclization: This is a common method involving treatment with a strong acid like p-toluenesulfonic acid (TsOH) or sulfuric acid (H₂SO₄). The acid protonates the hydroxyl group, making it a better leaving group, and facilitates the nucleophilic attack of the hydroxyl oxygen on the carbonyl carbon of the aldehyde (after deprotection).
-
Other methods: Other methods like using coupling reagents (e.g., DCC or EDCI) to activate the carboxylic acid (formed by oxidation of the aldehyde) can also be explored, though potentially less efficient for this specific transformation.
Reaction Scheme (Step 3):
OH O
| |
CH₃CH₂CH₂CH-CH(OR)₂ --[Acid-catalyzed cyclization & Deprotection] --> CH₃CH₂CH₂CH₂-C
|
O
|
CH₂
Optimization and Considerations
The success of this synthesis hinges on several factors:
-
Reagent selection: Choosing the right reducing agent and protecting group is critical for selectivity and yield. The compatibility of these reagents with each other and with the subsequent reactions should be carefully evaluated.
-
Reaction conditions: Reaction temperature, solvent, concentration, and reaction time all impact the efficiency and selectivity of each step. Optimization of these parameters is crucial.
-
Purification techniques: Effective purification strategies (e.g., chromatography, recrystallization) are essential to isolate the desired product and remove byproducts.
-
Yield and scalability: The overall yield of the synthesis should be optimized to maximize the amount of 4-hexanolide obtained. The scalability of the process for larger-scale production should also be considered.
Alternative Synthetic Routes
While the proposed route provides a plausible approach, alternative synthetic strategies can be explored. For instance, the use of different protecting groups, different cyclization methods or even starting from different precursors could provide alternative pathways. The specific choice will depend on several factors, including the availability of starting materials, cost considerations, and desired overall efficiency. A detailed literature search could uncover more specialized and efficient methods.
Conclusion
The synthesis of 4-hexanolide from 4-oxohexanal requires a multi-step approach that combines selective reduction, aldehyde protection, and efficient lactonization. The proposed three-step strategy utilizing NaBH₄ reduction, acetal protection, and acid-catalyzed cyclization provides a viable pathway. However, optimization of reaction conditions, reagent selection, and purification techniques are crucial for maximizing yield and efficiency. Exploring alternative synthetic routes and evaluating the literature for specialized methods are recommended to further refine this synthesis. This analysis highlights the iterative nature of synthetic organic chemistry, requiring careful planning, experimental execution, and thorough analysis to achieve the desired target molecule. Further research into specific reaction conditions and optimization is necessary to obtain a high-yielding and robust synthetic method.
Latest Posts
Latest Posts
-
You Are The Network Administrator For A Small Consulting Firm
May 09, 2025
-
A Separate Peace Chapter 6 Summary
May 09, 2025
-
Sculpture More Than Painting Appeals To
May 09, 2025
-
Extension Questions Model 3 Timing Of Dna Replication
May 09, 2025
-
A Biblical Worldview Is Rooted In Truth And Never Changes
May 09, 2025
Related Post
Thank you for visiting our website which covers about Design A Synthesis Of 4-hexanolide From 4-oxohexanal . We hope the information provided has been useful to you. Feel free to contact us if you have any questions or need further assistance. See you next time and don't miss to bookmark.