Exercise 13 Review Sheet Neuron Anatomy And Physiology
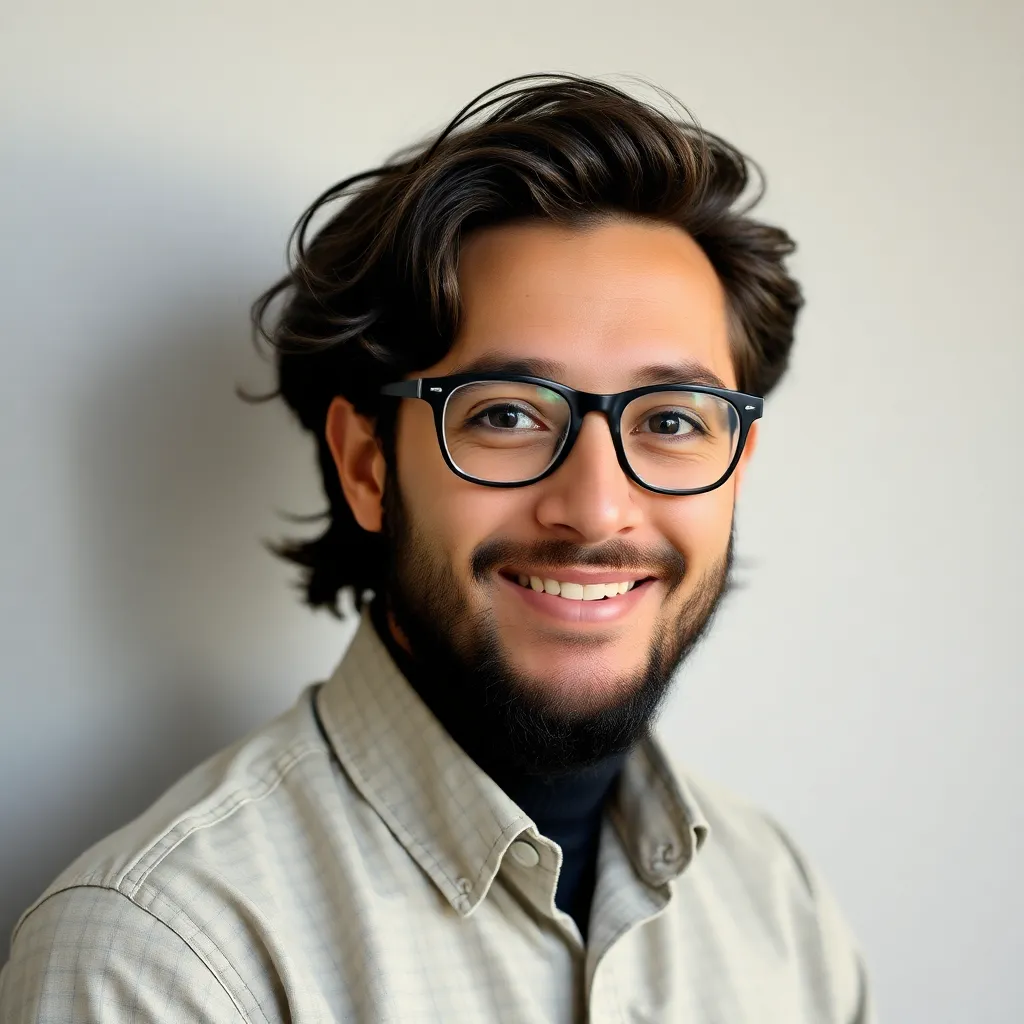
Onlines
Apr 18, 2025 · 9 min read

Table of Contents
Exercise 13 Review Sheet: Neuron Anatomy and Physiology
This comprehensive guide delves into the intricacies of neuron anatomy and physiology, providing a detailed review of key concepts crucial for understanding the nervous system. We'll explore the structure of neurons, their functional components, the electrochemical processes enabling communication, and the various types of neurons found throughout the body. This in-depth exploration will solidify your understanding of this vital subject.
I. Neuron Structure: The Building Blocks of the Nervous System
Neurons, the fundamental units of the nervous system, are highly specialized cells responsible for receiving, processing, and transmitting information. Understanding their structure is paramount to comprehending their function.
A. The Soma (Cell Body): The Neuron's Control Center
The soma, or cell body, is the neuron's metabolic center. It contains the nucleus, which houses the genetic material (DNA), and other essential organelles like mitochondria (responsible for energy production) and ribosomes (involved in protein synthesis). These organelles are crucial for maintaining the neuron's structure and function. The soma integrates signals received from dendrites and initiates the action potential.
B. Dendrites: Receiving Signals
Dendrites are branched extensions of the soma that act as the primary receivers of signals from other neurons. They are studded with specialized receptors that bind to neurotransmitters, chemical messengers released by other neurons. The more branching dendrites a neuron possesses, the more signals it can receive and integrate. The complexity of dendritic branching significantly influences neuronal processing and connectivity. The shape and structure of dendrites are highly variable, reflecting the diverse roles of different neuron types.
C. Axon: Transmitting Signals
The axon is a long, slender projection extending from the soma, responsible for transmitting signals over long distances. It is typically covered in a myelin sheath, a fatty insulating layer that significantly speeds up signal transmission. The myelin sheath is formed by glial cells (oligodendrocytes in the central nervous system and Schwann cells in the peripheral nervous system). Nodes of Ranvier, gaps in the myelin sheath, are crucial for saltatory conduction, a process that accelerates action potential propagation. The axon terminal, at the end of the axon, forms synapses with other neurons or target cells, allowing for signal transmission.
D. Myelin Sheath and Nodes of Ranvier: Facilitating Rapid Signal Transmission
The myelin sheath dramatically increases the speed of signal transmission along the axon. This insulation prevents ion leakage, allowing the action potential to "jump" between Nodes of Ranvier in a process called saltatory conduction. This process is significantly faster than continuous conduction in unmyelinated axons. Damage to the myelin sheath, as seen in diseases like multiple sclerosis, can severely impair neural transmission. The integrity of the myelin sheath is essential for proper nervous system function.
E. Axon Terminals (Synaptic Terminals): Communication Junctions
Axon terminals, also known as synaptic terminals or synaptic boutons, are specialized swellings at the end of axons. They form synapses with other neurons or effector cells (e.g., muscle cells, gland cells). These synapses are the sites of neurotransmitter release, enabling communication between neurons. The structure and organization of synapses are crucial for the precise transmission of neural signals. Different types of synapses exist, exhibiting varying degrees of efficacy and plasticity.
II. Neuron Physiology: The Electrochemical Language of the Nervous System
Neurons communicate through electrochemical signals, a complex interplay of electrical and chemical processes.
A. Resting Membrane Potential: Maintaining Polarity
The resting membrane potential is the electrical difference across the neuronal membrane when the neuron is at rest. This potential is typically around -70 mV, meaning the inside of the neuron is negatively charged relative to the outside. This negative charge is maintained by ion pumps and channels that selectively allow certain ions to cross the membrane. The sodium-potassium pump plays a crucial role, actively transporting sodium ions out of the cell and potassium ions into the cell, contributing significantly to the negative resting potential.
B. Action Potential: The Nerve Impulse
An action potential is a rapid, transient depolarization of the neuronal membrane. It is an all-or-none event, meaning it either occurs fully or not at all. The action potential is triggered when the membrane potential reaches a threshold level, typically around -55 mV. This depolarization is caused by a rapid influx of sodium ions into the cell, followed by a repolarization phase involving the efflux of potassium ions. The action potential propagates down the axon, transmitting the signal to the axon terminals. The speed of propagation is influenced by axon diameter and myelination.
C. Depolarization and Repolarization: The Two Phases of the Action Potential
Depolarization is the process by which the membrane potential becomes less negative, approaching zero. This is primarily due to the opening of voltage-gated sodium channels, allowing a rapid influx of sodium ions into the neuron. Repolarization is the process by which the membrane potential returns to its resting negative value. This is primarily due to the opening of voltage-gated potassium channels, allowing potassium ions to flow out of the neuron. These two phases are essential for the propagation of the action potential. The precise timing and magnitude of these changes are crucial for accurate neuronal signaling.
D. Refractory Period: Limiting Firing Rate
The refractory period is a brief period after an action potential during which the neuron is unable to generate another action potential. This period is essential for ensuring unidirectional propagation of the action potential and limiting the neuron's firing rate. The refractory period is divided into two phases: the absolute refractory period, during which no action potential can be generated, and the relative refractory period, during which an action potential can be generated only by a stronger-than-normal stimulus.
E. Synaptic Transmission: Chemical Communication
Synaptic transmission is the process by which signals are transmitted from one neuron to another across a synapse. This process involves the release of neurotransmitters from the presynaptic neuron, their diffusion across the synaptic cleft, and their binding to receptors on the postsynaptic neuron. The binding of neurotransmitters to receptors can either excite or inhibit the postsynaptic neuron, depending on the type of receptor and neurotransmitter involved. Synaptic transmission is a highly regulated process that is crucial for the complex processing of information within the nervous system.
F. Neurotransmitters: Chemical Messengers
Neurotransmitters are chemical messengers released by neurons to communicate with other neurons or effector cells. Different neurotransmitters have different effects on the postsynaptic neuron, mediating a wide range of neural functions. Examples of major neurotransmitters include acetylcholine, dopamine, serotonin, glutamate, and GABA. The actions of neurotransmitters are often modulated by other factors, including receptor subtypes and reuptake mechanisms. The precise balance of neurotransmitters is crucial for maintaining normal brain function. Imbalances in neurotransmitter systems are implicated in various neurological and psychiatric disorders.
G. Excitatory and Inhibitory Postsynaptic Potentials (EPSPs and IPSPs): Summation and Integration
Excitatory postsynaptic potentials (EPSPs) are depolarizations of the postsynaptic membrane, making it more likely to fire an action potential. Inhibitory postsynaptic potentials (IPSPs) are hyperpolarizations of the postsynaptic membrane, making it less likely to fire an action potential. The postsynaptic neuron integrates these EPSPs and IPSPs, summing their effects. If the sum of EPSPs exceeds the threshold potential, an action potential is generated. This integration process allows for complex information processing within the nervous system. The balance between excitation and inhibition is crucial for proper neuronal function.
III. Types of Neurons: Specialized Roles in the Nervous System
Neurons are classified into several types based on their structure and function.
A. Sensory Neurons (Afferent Neurons): Transmitting Information from the Periphery
Sensory neurons carry information from sensory receptors to the central nervous system (CNS). They detect stimuli such as light, sound, touch, temperature, and pain. These neurons are crucial for our perception of the external and internal environment. The peripheral processes of sensory neurons extend to sensory receptors, while their central processes project into the spinal cord or brain.
B. Motor Neurons (Efferent Neurons): Sending Signals to Effectors
Motor neurons carry information from the CNS to effector organs, such as muscles and glands. They initiate muscle contractions and gland secretions. Motor neurons play a vital role in movement, behavior, and homeostasis. The cell bodies of motor neurons are located in the CNS, and their axons extend to target muscles or glands. The neuromuscular junction, the synapse between a motor neuron and a muscle fiber, is a crucial site for the initiation of muscle contraction.
C. Interneurons: Integrating Information within the CNS
Interneurons are located entirely within the CNS and serve to integrate information between sensory and motor neurons. They play a critical role in processing information and generating complex responses. Interneurons are highly diverse in their structure and function, contributing to the complexity of neural circuits. They are crucial for coordinating the activities of different parts of the nervous system.
IV. Glial Cells: Supporting Roles in the Nervous System
Glial cells, often called neuroglia, are non-neuronal cells that provide structural and functional support to neurons. They are crucial for maintaining the health and proper functioning of the nervous system.
A. Astrocytes: Maintaining Homeostasis
Astrocytes are star-shaped glial cells that provide structural support to neurons, regulate the extracellular environment, and participate in the blood-brain barrier. They play a vital role in maintaining homeostasis within the nervous system. Astrocytes are involved in many aspects of neuronal function, including synaptic transmission and neurotransmitter reuptake.
B. Oligodendrocytes (CNS) and Schwann Cells (PNS): Myelinating Axons
Oligodendrocytes in the CNS and Schwann cells in the PNS produce myelin, a fatty insulating layer that surrounds axons and significantly speeds up signal transmission. Myelination is crucial for the efficient functioning of the nervous system. Damage to myelin, as in multiple sclerosis, can severely impair neural transmission.
C. Microglia: Immune Defense
Microglia are the immune cells of the CNS, acting as phagocytes to remove cellular debris and pathogens. They play a crucial role in maintaining the health and integrity of the nervous system. Microglia are involved in both innate and adaptive immune responses within the CNS.
This extensive review sheet provides a thorough overview of neuron anatomy and physiology. Remember that understanding these fundamental concepts is essential for comprehending the complex workings of the nervous system and its crucial role in bodily function. Further exploration of specific neurotransmitters, neural pathways, and diseases affecting the nervous system will further enhance your knowledge in this vital area of biology.
Latest Posts
Latest Posts
-
The Thing Around Your Neck Summary
Apr 19, 2025
-
Which Of The Following Is Not An Effect Of Testosterone
Apr 19, 2025
-
Enzymes How Do They Work Worksheet
Apr 19, 2025
-
Cpl Rice And Sgt Davis Are Attending The Joint Exercise
Apr 19, 2025
-
There Are 3 Teams Of 18 Employees
Apr 19, 2025
Related Post
Thank you for visiting our website which covers about Exercise 13 Review Sheet Neuron Anatomy And Physiology . We hope the information provided has been useful to you. Feel free to contact us if you have any questions or need further assistance. See you next time and don't miss to bookmark.