Experiment 13 The Geometrical Structure Of Molecules Answers
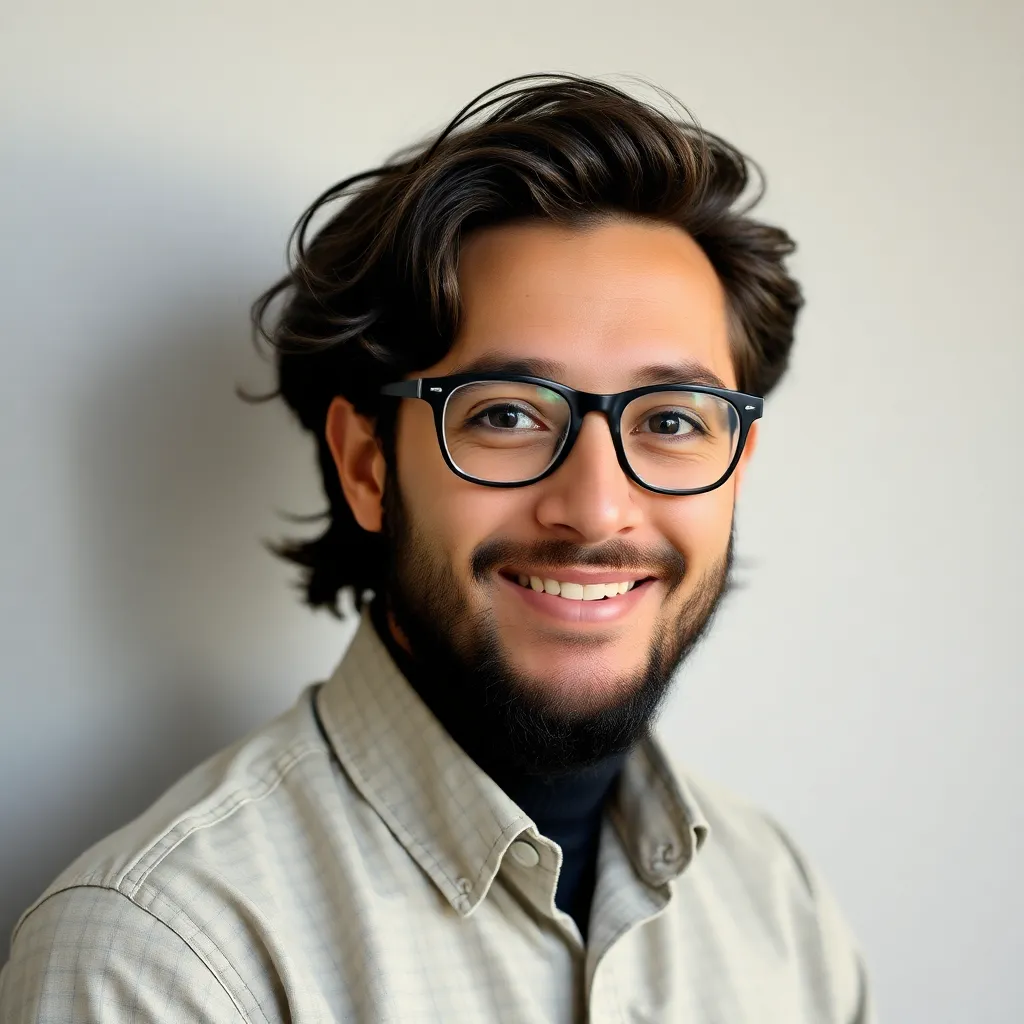
Onlines
Apr 01, 2025 · 6 min read
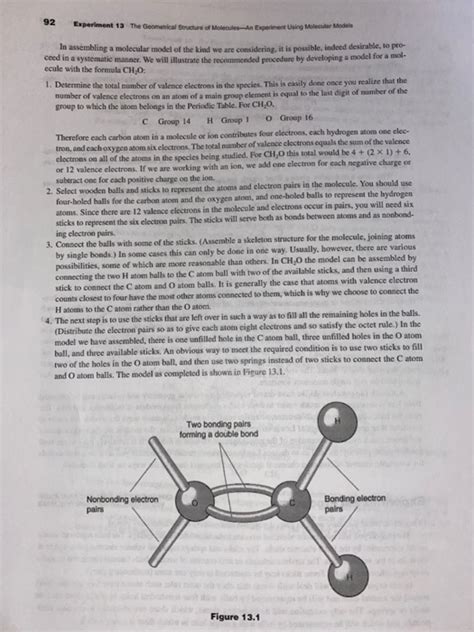
Table of Contents
- Experiment 13 The Geometrical Structure Of Molecules Answers
- Table of Contents
- Experiment 13: Unveiling the Geometrical Structures of Molecules – A Comprehensive Guide
- Understanding VSEPR Theory: The Foundation of Molecular Geometry
- Predicting Molecular Geometry: A Step-by-Step Approach
- Experiment 13: Common Molecules and Their Geometries
- Addressing Common Challenges and Misconceptions
- Beyond VSEPR: Advanced Concepts in Molecular Geometry
- Experiment 13: Data Analysis and Interpretation
- Conclusion: Mastering Molecular Geometry
- Latest Posts
- Latest Posts
- Related Post
Experiment 13: Unveiling the Geometrical Structures of Molecules – A Comprehensive Guide
Understanding the three-dimensional arrangement of atoms within a molecule, its molecular geometry, is crucial in chemistry. This geometry dictates a molecule's physical and chemical properties, influencing everything from its boiling point to its reactivity. Experiment 13, commonly found in introductory chemistry courses, aims to provide a hands-on understanding of molecular geometry using various models and prediction techniques. This detailed guide will walk you through the experiment, explain the underlying principles, and provide answers to common questions.
Understanding VSEPR Theory: The Foundation of Molecular Geometry
The Valence Shell Electron Pair Repulsion (VSEPR) theory is the cornerstone of predicting molecular geometries. This theory posits that electron pairs—both bonding and lone pairs—repel each other and will arrange themselves to minimize this repulsion. This arrangement ultimately determines the molecule's shape. The key to applying VSEPR theory lies in correctly identifying the number of electron domains surrounding the central atom. An electron domain can be a single bond, a double bond, a triple bond, or a lone pair of electrons.
Predicting Molecular Geometry: A Step-by-Step Approach
Let's break down the process of predicting molecular geometry using VSEPR theory:
-
Draw the Lewis Structure: This crucial first step involves determining the arrangement of atoms and valence electrons in the molecule. Remember to account for all valence electrons and satisfy the octet rule (or duet rule for hydrogen).
-
Count the Electron Domains: Identify the number of electron domains around the central atom. This includes both bonding pairs and lone pairs.
-
Determine the Electron Domain Geometry: Based on the number of electron domains, determine the electron domain geometry using the following table:
Number of Electron Domains | Electron Domain Geometry |
---|---|
2 | Linear |
3 | Trigonal Planar |
4 | Tetrahedral |
5 | Trigonal Bipyramidal |
6 | Octahedral |
- Identify the Molecular Geometry: This step accounts for the presence of lone pairs. Lone pairs occupy more space than bonding pairs, causing slight distortions in the molecular geometry. The molecular geometry describes the arrangement of atoms only. Here's a summary for common geometries:
For AX₂ type molecules (2 bonding pairs, 0 lone pairs): Linear
For AX₃ type molecules (3 bonding pairs, 0 lone pairs): Trigonal Planar
For AX₄ type molecules (4 bonding pairs, 0 lone pairs): Tetrahedral
For AX₂E type molecules (2 bonding pairs, 1 lone pair): Bent (or V-shaped)
For AX₃E type molecules (3 bonding pairs, 1 lone pair): Trigonal Pyramidal
For AX₂E₂ type molecules (2 bonding pairs, 2 lone pairs): Bent (or V-shaped)
For AX₅ type molecules (5 bonding pairs, 0 lone pairs): Trigonal Bipyramidal
For AX₄E type molecules (4 bonding pairs, 1 lone pair): Seesaw
For AX₃E₂ type molecules (3 bonding pairs, 2 lone pairs): T-shaped
For AX₂E₃ type molecules (2 bonding pairs, 3 lone pairs): Linear
For AX₆ type molecules (6 bonding pairs, 0 lone pairs): Octahedral
For AX₅E type molecules (5 bonding pairs, 1 lone pair): Square Pyramidal
For AX₄E₂ type molecules (4 bonding pairs, 2 lone pairs): Square Planar
- Use Molecular Models: Constructing physical models using molecular model kits provides a tangible representation of the predicted geometry, reinforcing your understanding. This allows for visualization of bond angles and spatial relationships between atoms.
Experiment 13: Common Molecules and Their Geometries
Experiment 13 typically involves analyzing several molecules using the VSEPR theory approach described above. Here are some common examples and their predicted geometries:
-
Water (H₂O): The central atom is oxygen. Oxygen has two bonding pairs and two lone pairs. The electron domain geometry is tetrahedral, but the molecular geometry is bent due to the lone pairs.
-
Ammonia (NH₃): Nitrogen is the central atom with three bonding pairs and one lone pair. The electron domain geometry is tetrahedral, resulting in a trigonal pyramidal molecular geometry.
-
Methane (CH₄): Carbon is the central atom with four bonding pairs and no lone pairs. The electron domain geometry and molecular geometry are both tetrahedral.
-
Carbon Dioxide (CO₂): Carbon is the central atom with two double bonds and no lone pairs. The electron domain geometry and molecular geometry are both linear.
-
Sulfur Hexafluoride (SF₆): Sulfur is the central atom surrounded by six fluorine atoms. The electron domain geometry and molecular geometry are both octahedral.
Addressing Common Challenges and Misconceptions
-
Distinguishing between electron domain geometry and molecular geometry: Remember that electron domain geometry considers all electron domains (bonding and lone pairs), while molecular geometry considers only the arrangement of atoms.
-
Accounting for multiple bonds: Double and triple bonds count as a single electron domain when applying VSEPR theory.
-
Understanding lone pair repulsion: Lone pairs repel each other more strongly than bonding pairs, leading to distortions in the molecular geometry.
-
Visualizing 3D structures: Using molecular models is crucial for understanding the three-dimensional nature of these molecules.
Beyond VSEPR: Advanced Concepts in Molecular Geometry
While VSEPR theory provides a simple and effective method for predicting molecular geometries, it's crucial to understand its limitations. More sophisticated techniques, such as Density Functional Theory (DFT) and ab initio calculations, can provide a more accurate description of molecular geometries, especially for larger and more complex molecules. These methods consider electron density distributions more precisely and can account for subtle interactions not considered in VSEPR.
Experiment 13: Data Analysis and Interpretation
After constructing models and predicting geometries, Experiment 13 often involves analyzing the data obtained through experimental techniques such as spectroscopy (infrared or Raman spectroscopy) or X-ray crystallography. These techniques provide experimental evidence confirming or refining the predicted geometries. Analyzing the data involves comparing the experimental bond lengths, bond angles, and vibrational frequencies to theoretical predictions based on the VSEPR model. Discrepancies could be due to limitations of the VSEPR theory, intermolecular interactions, or experimental error.
Conclusion: Mastering Molecular Geometry
Experiment 13 provides a valuable introduction to the concept of molecular geometry. By understanding and applying VSEPR theory, you can predict the three-dimensional arrangement of atoms in a molecule, a crucial step in understanding its properties and reactivity. Remember to practice, utilize molecular models, and be aware of the limitations of VSEPR theory to gain a robust understanding of this fundamental aspect of chemistry. While VSEPR is a powerful tool for predicting molecular geometries, it's essential to acknowledge its limitations and appreciate the sophistication of more advanced computational techniques used for precise geometrical determination. The combination of theory, modeling, and experimental analysis provides a holistic understanding of this important chemical concept. Through this detailed exploration of Experiment 13, you are well-equipped to navigate the fascinating world of molecular structures.
Latest Posts
Latest Posts
-
5 11 6 Spoof Mac Addresses With Smac
Apr 06, 2025
-
Which Of The Following Best Describes A Control Relay
Apr 06, 2025
-
Ati Capstone Leadership And Community Health Assessment
Apr 06, 2025
-
Summary Of Act 1 Of Othello
Apr 06, 2025
-
The Drawing Shows Three Polarizer Analyzer Pairs
Apr 06, 2025
Related Post
Thank you for visiting our website which covers about Experiment 13 The Geometrical Structure Of Molecules Answers . We hope the information provided has been useful to you. Feel free to contact us if you have any questions or need further assistance. See you next time and don't miss to bookmark.