For Each Compound Determine The Direction Of Bond Polarity
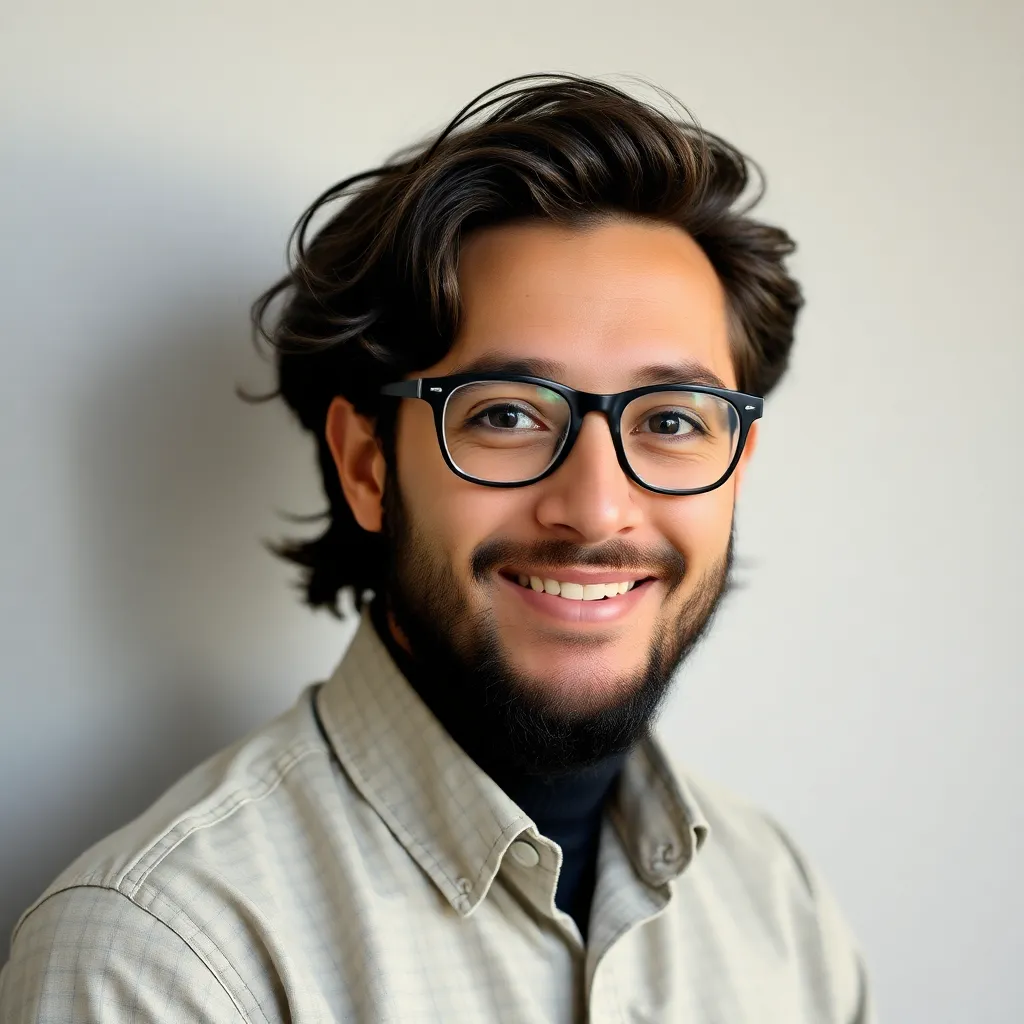
Onlines
May 08, 2025 · 5 min read
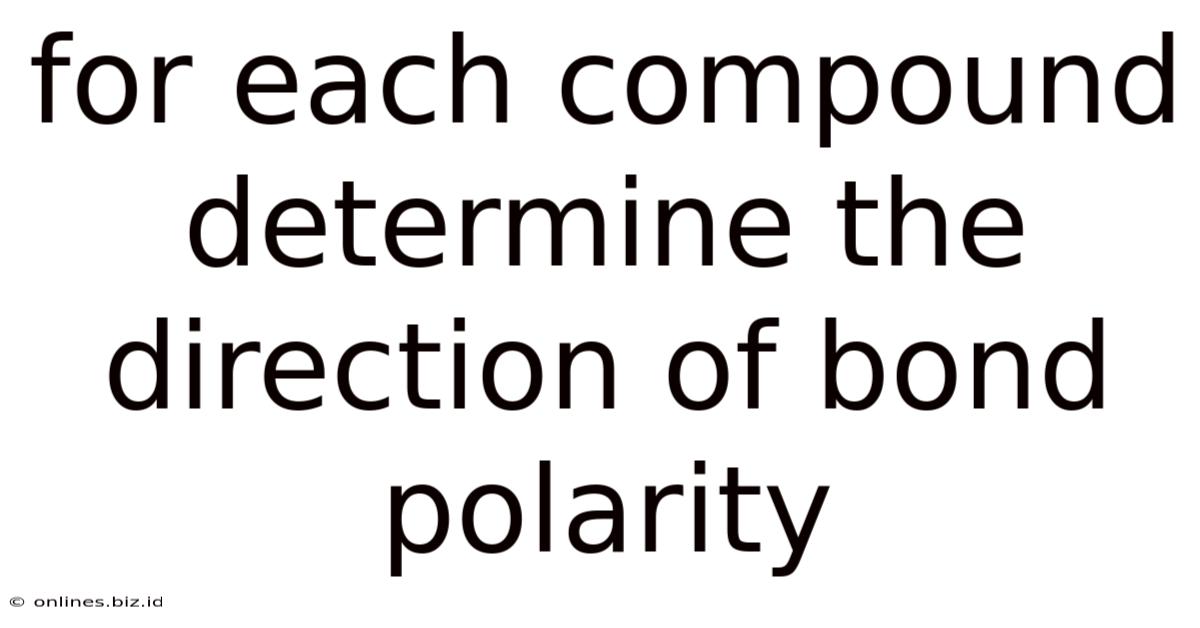
Table of Contents
Determining the Direction of Bond Polarity: A Comprehensive Guide
Understanding bond polarity is fundamental to comprehending the behavior of molecules. It dictates the distribution of electron density within a molecule, influencing its physical and chemical properties, including solubility, boiling point, and reactivity. This article provides a comprehensive guide to determining the direction of bond polarity for various compounds, focusing on electronegativity differences and the resulting dipole moments.
What is Bond Polarity?
A polar bond arises when two atoms with different electronegativities share electrons. Electronegativity, denoted by χ (chi), is a measure of an atom's ability to attract electrons within a chemical bond. The greater the difference in electronegativity between two atoms, the more polar the bond. In a perfectly nonpolar bond (like in a diatomic molecule of the same element, e.g., H₂ or O₂), the electrons are shared equally. Conversely, in a highly polar bond, the electrons are significantly closer to the more electronegative atom, creating a dipole moment.
The dipole moment (µ) is a vector quantity that measures the magnitude and direction of the bond polarity. It's represented by an arrow pointing from the less electronegative atom (positive end, δ+) to the more electronegative atom (negative end, δ-). The length of the arrow represents the magnitude of the dipole moment.
Factors Affecting Electronegativity
Several factors influence an atom's electronegativity:
- Nuclear Charge: Higher nuclear charge attracts electrons more strongly, increasing electronegativity.
- Atomic Radius: Smaller atomic radius leads to stronger attraction of electrons to the nucleus, increasing electronegativity.
- Shielding Effect: Inner electrons shield outer electrons from the full nuclear charge, reducing electronegativity.
Determining Bond Polarity: A Step-by-Step Approach
To determine the direction of bond polarity, follow these steps:
-
Identify the atoms involved in the bond.
-
Determine the electronegativity values of each atom. You can find electronegativity values on various periodic tables and chemistry resources. The Pauling scale is commonly used.
-
Calculate the electronegativity difference (Δχ): Subtract the electronegativity of the less electronegative atom from the electronegativity of the more electronegative atom. Δχ = χ(more electronegative) - χ(less electronegative).
-
Interpret the electronegativity difference:
- Δχ = 0: Nonpolar covalent bond (equal sharing of electrons).
- 0 < Δχ < 0.5: Slightly polar covalent bond (electrons are shared unequally but the difference is minimal).
- 0.5 < Δχ < 1.7: Polar covalent bond (significant unequal sharing of electrons).
- Δχ > 1.7: Ionic bond (electrons are essentially transferred from one atom to another).
-
Indicate the direction of the dipole moment: Draw an arrow from the less electronegative atom (δ+) to the more electronegative atom (δ-).
Examples of Determining Bond Polarity
Let's illustrate the process with several examples:
1. Hydrogen Chloride (HCl)
- Atoms: Hydrogen (H) and Chlorine (Cl).
- Electronegativity: χ(H) ≈ 2.2, χ(Cl) ≈ 3.2
- Electronegativity difference: Δχ = 3.2 - 2.2 = 1.0
- Interpretation: This is a polar covalent bond.
- Direction: The dipole moment points from H (δ+) to Cl (δ-).
2. Carbon Dioxide (CO₂)
- Atoms: Carbon (C) and Oxygen (O).
- Electronegativity: χ(C) ≈ 2.5, χ(O) ≈ 3.5
- Electronegativity difference: Δχ = 3.5 - 2.5 = 1.0 (for each C=O bond).
- Interpretation: Each C=O bond is polar covalent. However, the molecule is linear. The two dipole moments of the C=O bonds are equal in magnitude but opposite in direction, resulting in a net dipole moment of zero. Therefore, CO₂ is a nonpolar molecule despite having polar bonds.
3. Water (H₂O)
- Atoms: Hydrogen (H) and Oxygen (O).
- Electronegativity: χ(H) ≈ 2.2, χ(O) ≈ 3.5
- Electronegativity difference: Δχ = 3.5 - 2.2 = 1.3 (for each O-H bond).
- Interpretation: Each O-H bond is polar covalent. The bent molecular geometry of water means the dipole moments of the O-H bonds do not cancel each other out.
- Direction: The dipole moments of both O-H bonds contribute to a net dipole moment pointing towards the oxygen atom. Water is a polar molecule.
4. Methane (CH₄)
- Atoms: Carbon (C) and Hydrogen (H).
- Electronegativity: χ(C) ≈ 2.5, χ(H) ≈ 2.2
- Electronegativity difference: Δχ = 2.5 - 2.2 = 0.3 (for each C-H bond).
- Interpretation: Each C-H bond is slightly polar covalent. However, the tetrahedral geometry of methane causes the individual bond dipoles to cancel each other out, resulting in a nonpolar molecule.
5. Ammonia (NH₃)
- Atoms: Nitrogen (N) and Hydrogen (H).
- Electronegativity: χ(N) ≈ 3.0, χ(H) ≈ 2.2
- Electronegativity difference: Δχ = 3.0 - 2.2 = 0.8 (for each N-H bond).
- Interpretation: Each N-H bond is polar covalent. The pyramidal geometry of ammonia results in a net dipole moment pointing towards the nitrogen atom. Ammonia is a polar molecule.
Beyond Simple Diatomic Molecules: More Complex Scenarios
The principles outlined above apply to more complex molecules containing multiple bonds. For these molecules, you need to consider:
- Individual bond polarities: Determine the polarity of each bond within the molecule.
- Molecular geometry: The overall molecular shape significantly impacts the cancellation or summation of individual bond dipoles. Symmetrical molecules often have a net dipole moment of zero, even if individual bonds are polar.
- Vector addition of dipole moments: Treat the dipole moments as vectors and add them to determine the net dipole moment of the molecule.
Advanced Considerations: Resonance and Hybridization
In molecules with resonance structures or significant hybridization, the determination of bond polarity can be more nuanced. Resonance structures represent an average distribution of electrons, influencing the overall bond order and polarity. Hybridization affects the electron distribution around an atom, potentially altering the individual bond polarities. These aspects require a deeper understanding of advanced concepts in chemistry.
Conclusion
Determining the direction of bond polarity is a crucial skill in chemistry. By understanding electronegativity differences, molecular geometry, and the principles of dipole moments, you can accurately predict the polarity of various chemical bonds and molecules. This information is vital for understanding and predicting the physical and chemical properties of substances, laying the foundation for further studies in organic chemistry, physical chemistry, and biochemistry. Remember to always consider the interplay between individual bond polarities and the overall molecular structure when assessing the overall polarity of a molecule.
Latest Posts
Latest Posts
-
Match Each Amendment With Its Purpose
May 08, 2025
-
All Of The Following Cultures Were Mesopotamian Except
May 08, 2025
-
Gilded Age Dinner Party Answer Key
May 08, 2025
-
Consider A System Consisting Of Three Particles
May 08, 2025
-
A Company Has Unlimited Funds To Invest
May 08, 2025
Related Post
Thank you for visiting our website which covers about For Each Compound Determine The Direction Of Bond Polarity . We hope the information provided has been useful to you. Feel free to contact us if you have any questions or need further assistance. See you next time and don't miss to bookmark.