From The Following Compounds Involved In Cellular Respiration
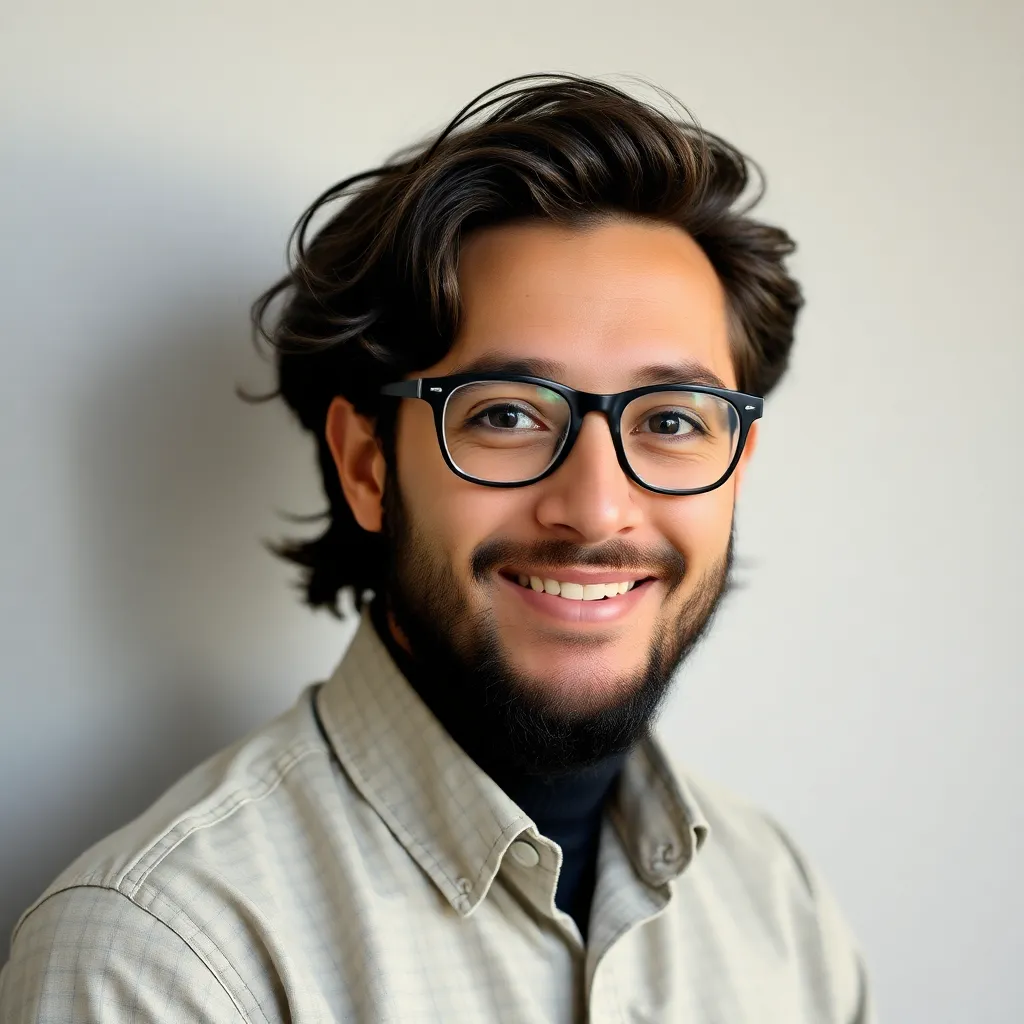
Onlines
May 10, 2025 · 6 min read
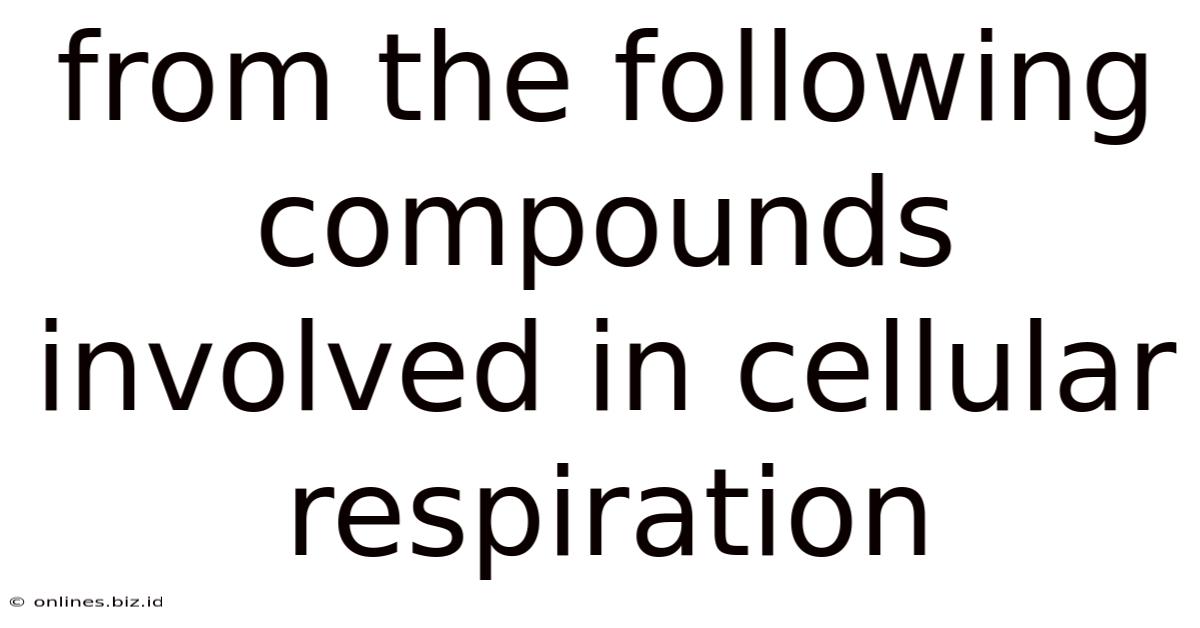
Table of Contents
From the Following Compounds Involved in Cellular Respiration: A Deep Dive into the Energy-Producing Processes of Life
Cellular respiration, the process by which cells break down glucose to produce ATP (adenosine triphosphate), the energy currency of life, is a complex and fascinating journey involving numerous compounds. This article delves into the key players in this intricate process, exploring their roles and interactions within glycolysis, the Krebs cycle (also known as the citric acid cycle), and the electron transport chain. Understanding these compounds is crucial to grasping the fundamental mechanisms that power all living organisms.
Stage 1: Glycolysis – Breaking Down Glucose
Glycolysis, meaning "sugar splitting," is the initial stage of cellular respiration, occurring in the cytoplasm of the cell. It doesn't require oxygen and is therefore considered anaerobic. This process involves a series of ten enzyme-catalyzed reactions that convert one molecule of glucose (a six-carbon sugar) into two molecules of pyruvate (a three-carbon compound). Let's examine the key compounds involved:
Key Compounds in Glycolysis:
- Glucose: The starting substrate, a six-carbon sugar derived from the breakdown of carbohydrates. It's the primary fuel source for glycolysis.
- Glucose-6-phosphate: The first intermediate formed when glucose is phosphorylated using ATP. This phosphorylation traps glucose within the cell and initiates the glycolytic pathway.
- Fructose-6-phosphate: Isomerization of glucose-6-phosphate, changing its structure to prepare for further reactions.
- Fructose-1,6-bisphosphate: A key regulatory point in glycolysis, further phosphorylated using another ATP molecule. This step commits the molecule to proceeding through the pathway.
- Glyceraldehyde-3-phosphate (G3P) and Dihydroxyacetone phosphate (DHAP): Fructose-1,6-bisphosphate splits into two three-carbon isomers, G3P and DHAP. DHAP is readily converted to G3P, ensuring that both molecules continue through the pathway.
- 1,3-Bisphosphoglycerate: Phosphorylation of G3P using inorganic phosphate (Pi). This is a crucial step, as it is subsequently used to generate ATP.
- 3-Phosphoglycerate: The high-energy phosphate bond in 1,3-bisphosphoglycerate is used to generate ATP through substrate-level phosphorylation.
- 2-Phosphoglycerate: Isomerization of 3-phosphoglycerate, shifting the phosphate group to prepare for the next step.
- Phosphoenolpyruvate (PEP): Dehydration of 2-phosphoglycerate, creating a high-energy phosphate bond crucial for ATP production.
- Pyruvate: The final product of glycolysis, a three-carbon compound. Its fate depends on the presence or absence of oxygen. In the presence of oxygen, pyruvate enters the mitochondria for further oxidation; in its absence, it undergoes fermentation.
- ATP and NADH: Glycolysis produces a net gain of 2 ATP molecules and 2 NADH molecules per glucose molecule. ATP provides immediate energy, while NADH carries electrons to the electron transport chain for later ATP production.
Stage 2: The Krebs Cycle (Citric Acid Cycle) – Oxidizing Pyruvate
If oxygen is present, pyruvate enters the mitochondria, the powerhouse of the cell. Here, it undergoes oxidative decarboxylation, converting into acetyl-CoA, which then enters the Krebs cycle. This cycle, a series of eight enzyme-catalyzed reactions, takes place in the mitochondrial matrix. It completes the oxidation of glucose, generating high-energy electron carriers that fuel the electron transport chain.
Key Compounds in the Krebs Cycle:
- Acetyl-CoA: Derived from pyruvate, this two-carbon molecule enters the Krebs cycle by combining with oxaloacetate (a four-carbon compound).
- Citrate: The six-carbon compound formed when acetyl-CoA combines with oxaloacetate. This initiates the cycle.
- Isocitrate: Isomerization of citrate, preparing it for the next oxidation step.
- α-Ketoglutarate: Oxidative decarboxylation of isocitrate, producing a five-carbon compound and releasing CO2. NADH is also produced.
- Succinyl-CoA: Further oxidation of α-ketoglutarate, producing a four-carbon compound linked to CoA. NADH and GTP (guanosine triphosphate – equivalent to ATP) are produced.
- Succinate: Conversion of succinyl-CoA to succinate, releasing CoA.
- Fumarate: Oxidation of succinate, producing FADH2 (another electron carrier).
- Malate: Hydration of fumarate, adding a water molecule.
- Oxaloacetate: Oxidation of malate, regenerating the four-carbon molecule that can accept another acetyl-CoA, thus completing the cycle.
- NADH, FADH2, GTP/ATP, and CO2: The Krebs cycle produces 3 NADH, 1 FADH2, 1 GTP (equivalent to ATP), and 2 CO2 molecules per acetyl-CoA molecule.
Stage 3: The Electron Transport Chain (ETC) – Generating ATP via Oxidative Phosphorylation
The electron transport chain, located in the inner mitochondrial membrane, is the final stage of cellular respiration. The high-energy electron carriers, NADH and FADH2, generated during glycolysis and the Krebs cycle, deliver their electrons to a series of protein complexes embedded in the membrane. As electrons move down the chain, energy is released and used to pump protons (H+) from the mitochondrial matrix to the intermembrane space, creating a proton gradient.
Key Compounds and Components in the ETC:
- NADH and FADH2: Electron carriers delivering high-energy electrons to the ETC.
- Electron Transport Chain Complexes (I-IV): A series of protein complexes that transfer electrons, gradually decreasing their energy level. Complex I and II receive electrons from NADH and FADH2, respectively.
- Ubiquinone (Coenzyme Q): A mobile electron carrier that shuttles electrons between complexes.
- Cytochrome c: Another mobile electron carrier, transferring electrons between complex III and complex IV.
- Oxygen (O2): The final electron acceptor in the chain. It combines with electrons and protons to form water (H2O).
- ATP Synthase: An enzyme that uses the proton gradient generated by the ETC to synthesize ATP. This process is called chemiosmosis or oxidative phosphorylation.
- ATP: The final energy product of cellular respiration, providing energy for cellular processes. The vast majority of ATP produced during cellular respiration is generated through oxidative phosphorylation. The proton motive force drives ATP synthesis through ATP synthase.
Regulation of Cellular Respiration
Cellular respiration is a highly regulated process, ensuring that energy production matches the cell's energy demands. Regulation occurs at various points, including:
- Glycolysis: Phosphofructokinase is a key regulatory enzyme, sensitive to levels of ATP, ADP, and citrate. High ATP levels inhibit the enzyme, slowing down glycolysis.
- Krebs Cycle: Citrate synthase, isocitrate dehydrogenase, and α-ketoglutarate dehydrogenase are key regulatory enzymes, sensitive to levels of ATP, NADH, and other metabolites.
- Electron Transport Chain: The rate of electron flow through the ETC is regulated by the availability of oxygen and the redox state of electron carriers.
Cellular Respiration and Other Metabolic Pathways
Cellular respiration is intricately linked to other metabolic pathways within the cell. For example:
- Gluconeogenesis: The synthesis of glucose from non-carbohydrate sources, often utilizing intermediates from the Krebs cycle.
- Fatty Acid Oxidation (β-oxidation): The breakdown of fatty acids into acetyl-CoA, feeding into the Krebs cycle.
- Amino Acid Catabolism: The breakdown of amino acids, with some producing intermediates that enter glycolysis or the Krebs cycle.
Understanding the intricate network of metabolic pathways and the key compounds involved is essential for appreciating the remarkable efficiency and complexity of cellular energy production. The interplay of these pathways allows cells to adapt to changing energy demands and utilize a variety of fuel sources to maintain life's processes. Further research continues to uncover the nuanced details of cellular respiration and its role in health and disease.
Latest Posts
Latest Posts
-
Animals Eliminate Extra Carbon Dioxide By
May 10, 2025
-
In A Commercial Building Wires Are Typically Installed In
May 10, 2025
-
Physical Science If8767 Answer Key Page 61
May 10, 2025
-
Can You Get 5 Cash Back At Family Dollar
May 10, 2025
-
One Advantage Of The Glass Stem Thermometer Is That It
May 10, 2025
Related Post
Thank you for visiting our website which covers about From The Following Compounds Involved In Cellular Respiration . We hope the information provided has been useful to you. Feel free to contact us if you have any questions or need further assistance. See you next time and don't miss to bookmark.