How Do We Measure And Define Evolution
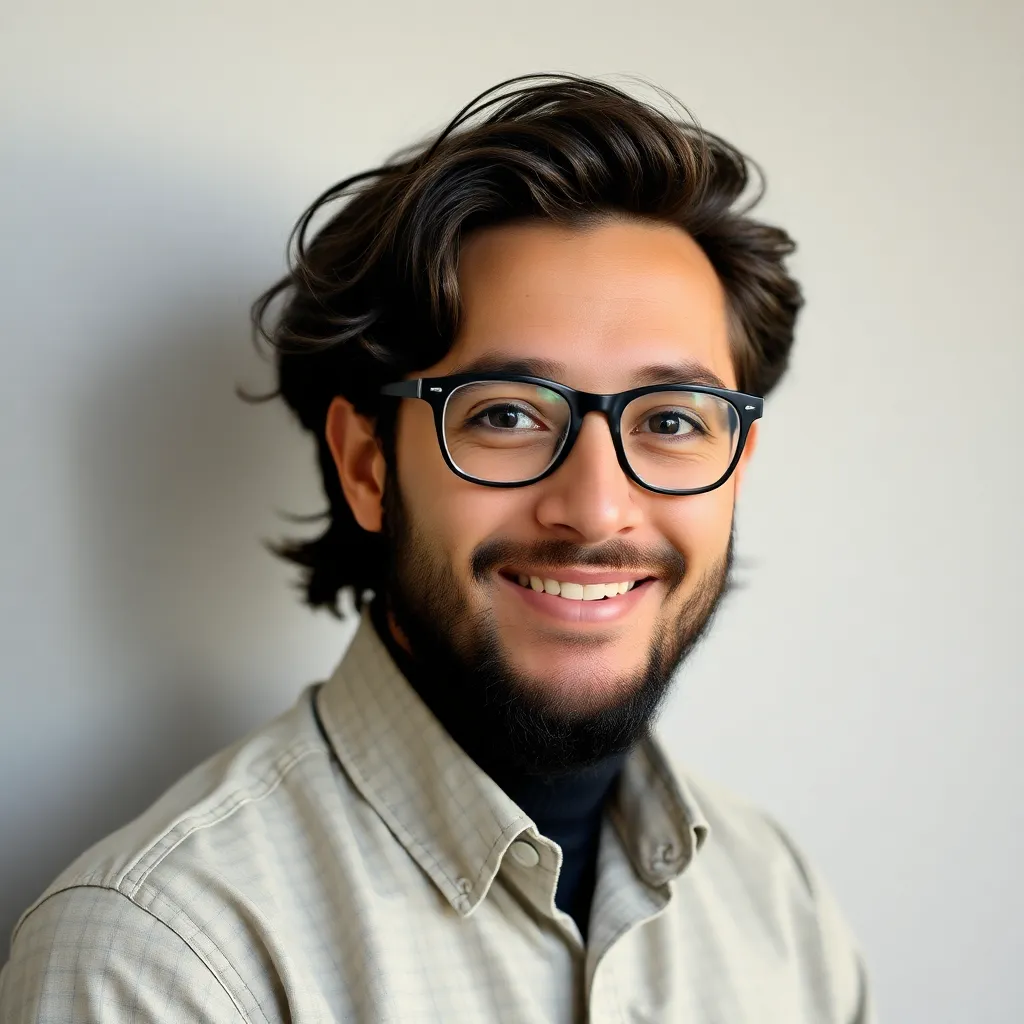
Onlines
May 07, 2025 · 6 min read
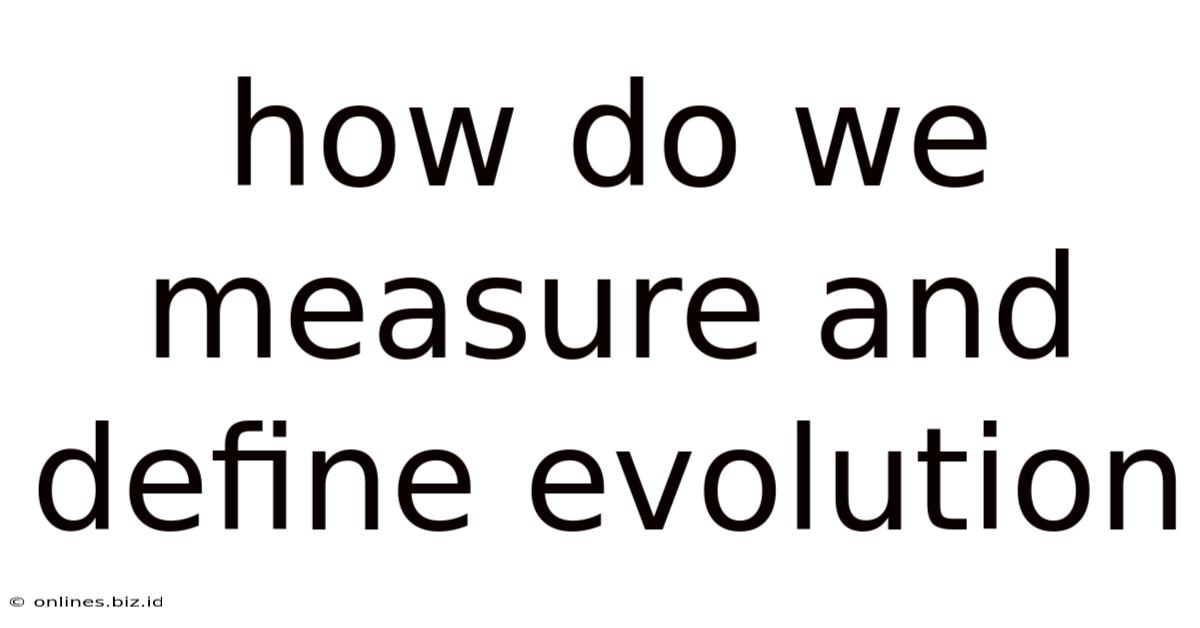
Table of Contents
How Do We Measure and Define Evolution?
Evolution, the cornerstone of modern biology, is the change in the heritable characteristics of biological populations over successive generations. This seemingly simple definition, however, belies the complex processes and multifaceted measurements used to understand and quantify this fundamental process. Measuring and defining evolution requires a multi-pronged approach, integrating evidence from various disciplines like genetics, paleontology, and comparative anatomy. This article will delve into the methods scientists employ to understand and quantify this transformative process.
Defining Evolution: Beyond Simple Change
While often simplified as "change over time," a rigorous definition of evolution necessitates specifying the type of change. It's not simply any change – for example, a change in the average height of individuals in a population due to improved nutrition isn't considered evolution unless that change is heritable (passed down through genes to offspring). Therefore, the core of evolution lies in alterations to the genetic makeup of a population, leading to changes in observable traits (phenotypes). These genetic changes are driven by mechanisms like mutation, genetic drift, gene flow, and natural selection.
The Role of Heritability
The critical element distinguishing evolutionary change from other types of change is heritability. Heritable traits are encoded within an organism's genes and can be passed from parents to their offspring. Non-heritable changes, such as those caused by environmental factors affecting an individual's phenotype during its lifetime, are not considered part of evolution. For example, a plant growing taller due to increased sunlight is a phenotypic change, but not an evolutionary one unless the increased height is due to a genetic change that increases the plant's ability to grow taller in sunny conditions and is passed to offspring.
Mechanisms of Evolutionary Change
Several mechanisms drive evolutionary change. Understanding these mechanisms is crucial for accurately measuring and defining evolutionary processes:
-
Mutation: Random changes in an organism's DNA sequence are the ultimate source of new genetic variation. These mutations can be beneficial, neutral, or harmful, and their impact on evolution depends on their heritability and effect on reproductive success.
-
Genetic Drift: This is a random change in allele frequencies within a population, particularly pronounced in small populations. Genetic drift can lead to the loss of beneficial alleles and the fixation of harmful ones, significantly affecting the genetic makeup of a population.
-
Gene Flow: The movement of genes between populations through migration and interbreeding can alter the genetic composition of both populations involved. Gene flow can introduce new genetic variation or homogenize genetic differences between populations.
-
Natural Selection: The differential survival and reproduction of individuals based on their traits. Individuals with traits better suited to their environment tend to leave more offspring, thus increasing the frequency of those beneficial traits in future generations. This is a non-random process that can lead to significant adaptive changes.
Measuring Evolution: Evidence and Techniques
Measuring evolution involves assessing changes in the genetic makeup and observable traits of populations over time. This requires a combination of approaches:
1. Fossil Record: A Window to the Past
Paleontology provides invaluable evidence of evolutionary change over vast timescales. The fossil record, while incomplete, documents the existence of extinct species and reveals transitional forms, showing how organisms changed over millions of years. Analyzing the morphology (physical form) of fossils allows researchers to trace evolutionary lineages and infer evolutionary relationships.
Measuring Changes in Fossil Morphology
Quantitative methods are employed to analyze changes in fossil morphology across time. This includes:
-
Morphometrics: Measuring the size and shape of bones and other structures to identify trends in evolutionary changes. Geometric morphometrics, a sophisticated technique, allows for the analysis of complex shapes.
-
Phylogenetic Analysis: Constructing evolutionary trees (phylogenies) based on shared characteristics to infer evolutionary relationships and track changes through time. This helps researchers understand the evolutionary pathways taken by different groups of organisms.
2. Comparative Anatomy: Homologous and Analogous Structures
Comparing the anatomical structures of different species reveals insights into evolutionary relationships. Homologous structures, similar structures in different species inherited from a common ancestor (e.g., the forelimbs of vertebrates), indicate common ancestry and evolutionary divergence. Analogous structures, similar structures in different species that evolved independently due to similar selective pressures (e.g., wings of birds and insects), illustrate convergent evolution. The study of homologous structures provides strong evidence for common descent and helps measure the degree of evolutionary divergence.
3. Molecular Biology: Genetic Changes Over Time
Molecular biology provides the most direct way to measure evolutionary changes. By analyzing DNA and protein sequences, researchers can:
-
Measure genetic divergence: Comparing the DNA sequences of different species to quantify the degree of genetic difference between them. Greater genetic divergence generally indicates more extensive evolutionary divergence.
-
Track the spread of mutations: Identifying the frequency of specific mutations within and between populations over time can reveal the rate and patterns of evolutionary change.
-
Study gene expression: Investigating changes in the expression of genes under different conditions reveals insights into adaptive evolutionary changes.
Specific Molecular Techniques
-
DNA sequencing: Determining the precise order of nucleotides in a DNA molecule to identify mutations and compare sequences.
-
Phylogenetic analysis of DNA: Constructing evolutionary trees based on DNA sequences to infer evolutionary relationships.
-
Population genetics: Studying the genetic variation within and between populations to identify the factors driving evolutionary change.
4. Observational Studies: Evolution in Action
Evolution is not solely a process confined to the distant past. Observational studies allow us to witness evolutionary changes in real-time. Examples include:
-
Antibiotic resistance in bacteria: The rapid evolution of antibiotic resistance in bacterial populations is a dramatic example of natural selection in action.
-
Evolution of pesticide resistance in insects: Similar to antibiotic resistance, the evolution of pesticide resistance in insects highlights the speed and power of natural selection.
-
Evolutionary changes in response to environmental changes: Studying the responses of populations to environmental stressors, such as climate change, allows us to observe evolution in action and predict future changes.
Defining the "Rate" of Evolution
The rate of evolution varies considerably depending on several factors, including:
-
Generation time: Species with shorter generation times typically evolve faster than species with longer generation times.
-
Environmental changes: Rapid environmental changes often accelerate evolutionary change.
-
Strength of natural selection: Strong selective pressures lead to faster evolutionary change.
-
Genetic variation: Populations with high levels of genetic variation have greater potential for evolutionary change.
Measuring the rate of evolution involves quantifying the change in allele frequencies or phenotypic traits over a given time period. This can be expressed as a rate of change per generation or per unit of time. However, accurately estimating the rate of evolution often poses significant challenges, due to the complexity of evolutionary processes and the difficulty in tracking all relevant changes.
Conclusion: A Holistic Approach to Understanding Evolution
Measuring and defining evolution is a complex endeavor requiring an integrated approach, combining data from diverse fields. The fossil record, comparative anatomy, molecular biology, and observational studies all contribute to our understanding of this fundamental biological process. While the concept of evolution might appear simple in principle – change in heritable characteristics over time – its precise measurement and the identification of its driving forces requires sophisticated methodologies and an appreciation of the multifaceted nature of biological systems. Continued advancements in technology and analytical methods will further refine our ability to measure and define evolution, leading to a more complete understanding of the history of life on Earth.
Latest Posts
Latest Posts
-
A Smart Thermostat Can Accomplish Which Of The Following
May 08, 2025
-
Bile Assists In The Chemical Digestion Of Triglycerides By
May 08, 2025
-
Assistive Devices For Total Hip Arthroplasty Ati
May 08, 2025
-
Which Of The Graphs In The Figure Illustrates Hookes Law
May 08, 2025
-
What Percentage Of Cj Bacteria Are Resistant To Fq
May 08, 2025
Related Post
Thank you for visiting our website which covers about How Do We Measure And Define Evolution . We hope the information provided has been useful to you. Feel free to contact us if you have any questions or need further assistance. See you next time and don't miss to bookmark.