Identify Reagents That Can Be Used For The Following Synthesis
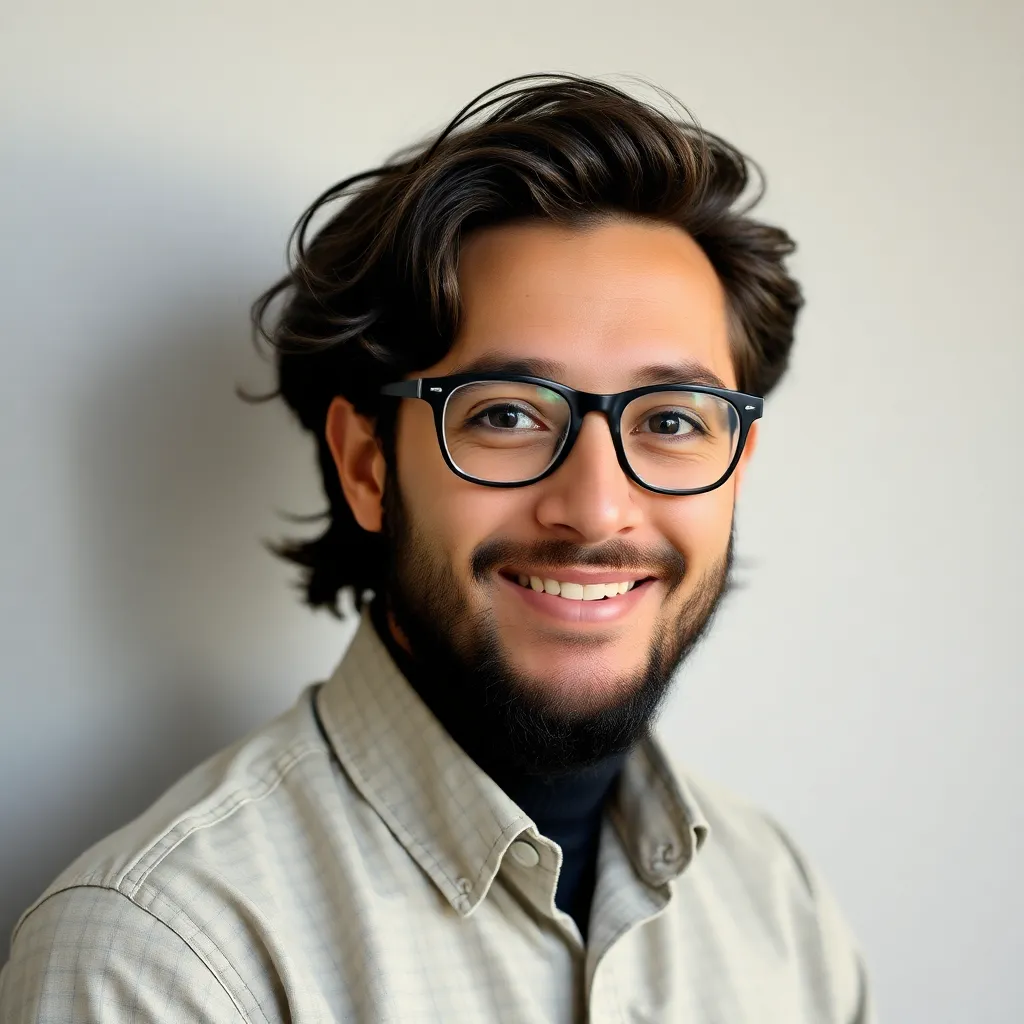
Onlines
May 10, 2025 · 6 min read
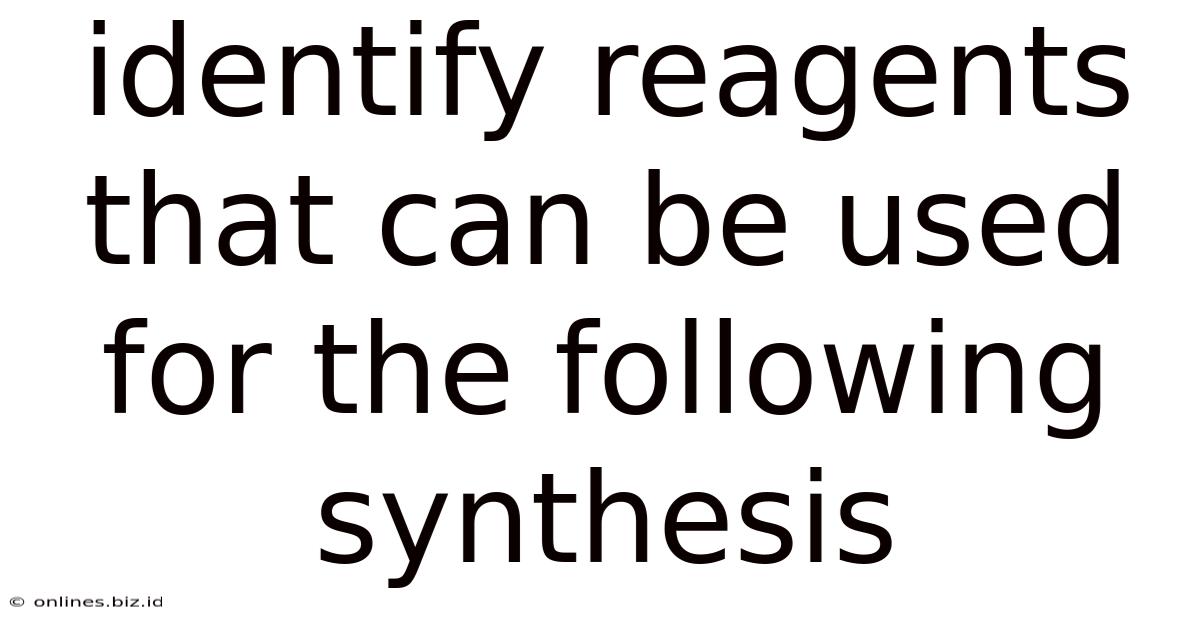
Table of Contents
Identifying Reagents for Organic Synthesis: A Comprehensive Guide
Organic synthesis, the art and science of constructing complex organic molecules from simpler precursors, relies heavily on the judicious selection of reagents. Choosing the right reagent is crucial for achieving high yields, selectivity, and minimizing the formation of unwanted byproducts. This article delves into the identification of reagents suitable for various synthetic transformations, providing a comprehensive overview of common reaction types and the reagents often employed.
Understanding Reagent Selection: Key Considerations
Before diving into specific examples, it’s vital to understand the factors that influence reagent selection. These include:
1. Functional Group Transformation:
The primary goal is often to convert one functional group into another. Understanding the reactivity of different functional groups and their susceptibility to various reagents is paramount. For example, converting an alcohol to a halide might require different reagents than converting a carboxylic acid to an amide.
2. Reaction Conditions:
Temperature, solvent, and pressure significantly impact reaction outcomes. Some reagents are sensitive to moisture or air, requiring anhydrous conditions. Others might require specific solvents to enhance solubility or reactivity.
3. Selectivity:
Ideally, a reagent should react selectively with a specific functional group in the presence of others. This is crucial in complex molecules where multiple functional groups might be present. Regioselectivity (reacting at a specific position on a molecule) and stereoselectivity (controlling the stereochemistry of the product) are key aspects of selectivity.
4. Yield and Purity:
The efficiency of a reagent is measured by its ability to produce the desired product in high yield and high purity. A reagent that provides a high yield with minimal byproducts is generally preferred.
5. Cost and Availability:
Economic factors and the accessibility of reagents also play a significant role in their selection. While some reagents might offer superior performance, their high cost or limited availability might necessitate the use of alternative, more readily accessible options.
6. Safety and Environmental Impact:
Safety is of paramount importance. Some reagents are highly toxic, corrosive, or flammable. Moreover, the environmental impact of reagents, particularly their toxicity and degradability, is increasingly considered in modern organic synthesis. Green chemistry principles emphasize the use of safer and more environmentally benign reagents.
Reagents for Common Synthetic Transformations:
Let’s examine various common organic transformations and the reagents typically employed:
1. Oxidation Reactions:
Oxidation reactions involve the increase in the oxidation state of a molecule. Common reagents for oxidation include:
-
Potassium permanganate (KMnO₄): A strong oxidizing agent capable of oxidizing alcohols to carboxylic acids, alkenes to diols, and even alkyl groups to carboxylic acids. Its strong oxidizing power necessitates careful control of reaction conditions.
-
Jones reagent (CrO₃/H₂SO₄): Effectively oxidizes primary alcohols to carboxylic acids and secondary alcohols to ketones. It's a powerful oxidant but generates chromium waste, raising environmental concerns.
-
Pyridinium chlorochromate (PCC): A milder oxidizing agent compared to Jones reagent, typically oxidizing primary alcohols to aldehydes and secondary alcohols to ketones. It offers better selectivity than stronger oxidants.
-
Dess-Martin periodinane (DMP): A highly selective and efficient reagent for oxidizing primary alcohols to aldehydes and secondary alcohols to ketones. It's generally preferred for sensitive substrates due to its mild conditions.
-
Swern oxidation: Uses DMSO, oxalyl chloride, and a base to oxidize alcohols to aldehydes or ketones. It's known for its mildness and high selectivity, especially useful for sensitive substrates.
2. Reduction Reactions:
Reduction reactions involve the decrease in the oxidation state of a molecule. Key reducing agents include:
-
Lithium aluminum hydride (LiAlH₄): A powerful reducing agent capable of reducing a wide range of functional groups, including esters, carboxylic acids, ketones, and aldehydes to alcohols. It's highly reactive and requires anhydrous conditions.
-
Sodium borohydride (NaBH₄): A milder reducing agent compared to LiAlH₄, primarily used for the reduction of aldehydes and ketones to alcohols. It's less reactive than LiAlH₄ and can be used in protic solvents.
-
Diborane (B₂H₆): Reduces carboxylic acids and esters to alcohols. It's a highly reactive gas and requires careful handling.
-
Catalytic hydrogenation (H₂/catalyst): Uses hydrogen gas in the presence of a metal catalyst (e.g., Pd/C, Pt/C, Ni) to reduce alkenes, alkynes, and other unsaturated functional groups. It's a widely used and versatile reduction method.
-
Zinc borohydride (Zn(BH₄)₂): A selective reducing agent that can reduce aldehydes and ketones in the presence of other functional groups, such as esters.
3. Grignard Reactions:
Grignard reagents (RMgX, where R is an alkyl or aryl group and X is a halide) are powerful nucleophiles used to form new carbon-carbon bonds. They react with carbonyl compounds (aldehydes, ketones, esters, etc.) to form alcohols.
-
Preparation of Grignard Reagents: Requires an alkyl or aryl halide and magnesium metal in anhydrous ether or THF. The reaction is highly sensitive to moisture and air.
-
Reactions of Grignard Reagents: The addition of a Grignard reagent to a carbonyl compound forms an alkoxide intermediate, which is then protonated to yield an alcohol. The type of carbonyl compound used dictates the type of alcohol formed (primary, secondary, or tertiary).
4. Wittig Reaction:
The Wittig reaction is a powerful method for converting aldehydes and ketones to alkenes. It utilizes a phosphorus ylide (a neutral molecule with a negatively charged carbon atom adjacent to a positively charged phosphorus atom) as a reagent.
-
Preparation of Ylides: Usually involves the reaction of a phosphonium salt with a strong base such as butyllithium.
-
Reaction with Aldehydes/Ketones: The ylide attacks the carbonyl carbon, forming a four-membered ring intermediate that subsequently collapses to form an alkene and a phosphine oxide.
5. Alkylation Reactions:
Alkylation reactions involve the addition of an alkyl group to a molecule. Common alkylating agents include:
-
Alkyl halides: React with nucleophiles (e.g., Grignard reagents, organolithium reagents, alkoxides) to form new carbon-carbon bonds.
-
Diazoalkanes: React with carboxylic acids to form esters.
-
Alkyl sulfonates: Similar reactivity to alkyl halides but generally more reactive.
6. Acylation Reactions:
Acylation reactions involve the addition of an acyl group (RCO-) to a molecule. Common acylating agents include:
-
Acid chlorides: React with alcohols to form esters, with amines to form amides.
-
Acid anhydrides: Similar reactivity to acid chlorides but generally less reactive.
-
Esters: Can undergo transesterification reactions to form different esters.
7. Esterification Reactions:
Esterification involves the reaction of a carboxylic acid with an alcohol to form an ester. Common reagents include:
- Acid catalysts: Such as sulfuric acid or p-toluenesulfonic acid, are often used to catalyze the reaction.
8. Amide Formation:
Amide formation involves the reaction of a carboxylic acid or its derivative (e.g., acid chloride, anhydride) with an amine. Common reagents include:
- Coupling reagents: Such as DCC (dicyclohexylcarbodiimide) or HATU (1-[Bis(dimethylamino)methylene]-1H-1,2,3-triazolo[4,5-b]pyridinium 3-oxid hexafluorophosphate), are frequently employed to activate the carboxylic acid and facilitate amide bond formation.
Conclusion:
Reagent selection in organic synthesis is a multifaceted process that requires a deep understanding of reaction mechanisms, functional group reactivity, and the specific requirements of the desired transformation. The examples provided here represent a fraction of the vast array of reagents available to organic chemists. Careful consideration of factors such as selectivity, yield, cost, safety, and environmental impact is crucial for choosing the most appropriate reagent for any given synthesis. Further exploration of specific reaction types and advanced techniques will reveal a wider range of reagents and their applications in the field of organic chemistry. The ability to effectively choose and utilize these reagents is fundamental to success in organic synthesis.
Latest Posts
Latest Posts
-
A Directory Is A Type Of File
May 10, 2025
-
An Office File Plan Is Not Required
May 10, 2025
-
For The Reaction Shown Select The Expected Major Organic Product
May 10, 2025
-
Letter Of Recommendation National Junior Honor Society
May 10, 2025
-
And Then There Were None Symbols
May 10, 2025
Related Post
Thank you for visiting our website which covers about Identify Reagents That Can Be Used For The Following Synthesis . We hope the information provided has been useful to you. Feel free to contact us if you have any questions or need further assistance. See you next time and don't miss to bookmark.