Investigation Dna Proteins And Sickle Cell
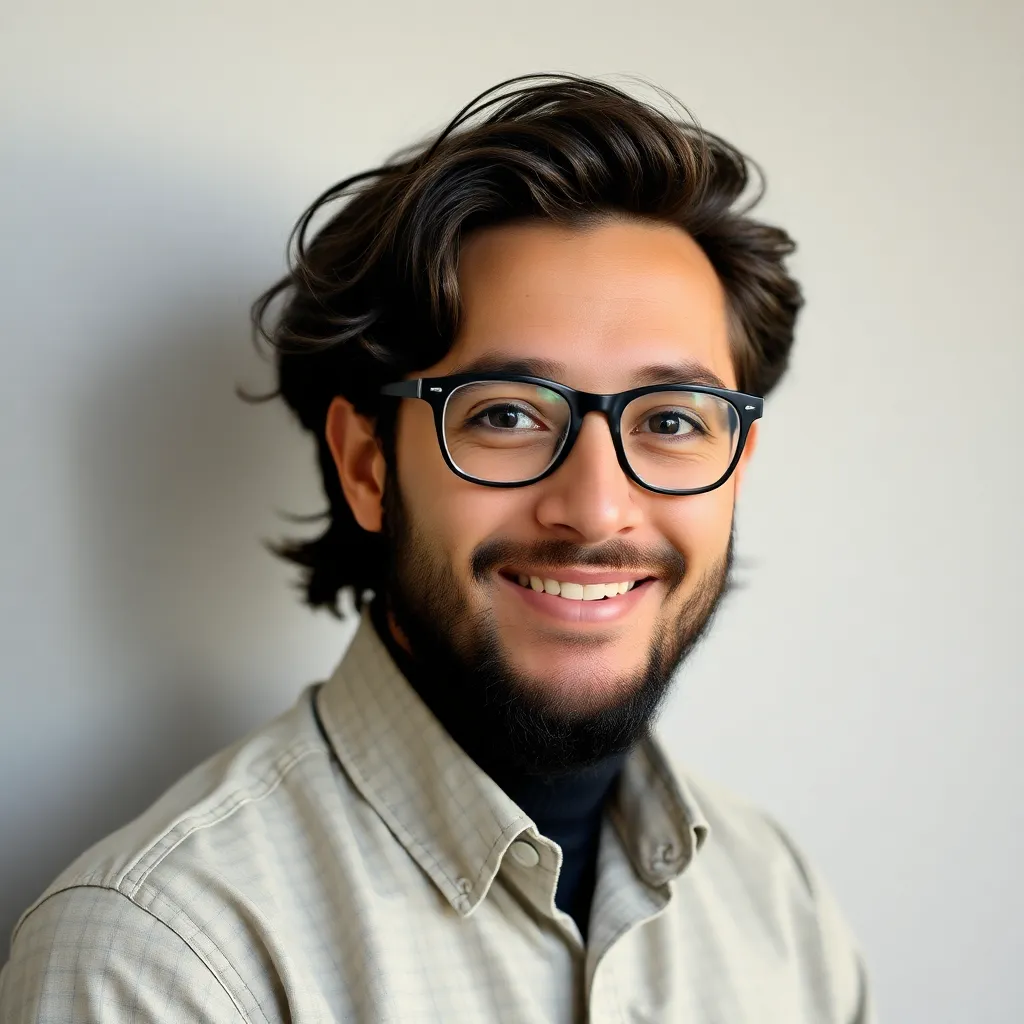
Onlines
Apr 22, 2025 · 6 min read

Table of Contents
Investigating DNA, Proteins, and Sickle Cell Disease: A Deep Dive
Sickle cell disease (SCD) stands as a compelling example of how a single alteration in DNA can profoundly impact protein structure and function, leading to a cascade of debilitating health consequences. Understanding SCD requires a journey through the intricate world of DNA, its transcription into RNA, translation into proteins, and the ultimate impact of protein misfolding on cellular processes. This comprehensive exploration delves into the molecular mechanisms underlying SCD, highlighting the interconnectedness of DNA, proteins, and the resultant disease phenotype.
From Genes to Proteins: The Central Dogma of Molecular Biology
At the heart of SCD lies a mutation in the gene encoding hemoglobin, the protein responsible for carrying oxygen in red blood cells. This gene, HBB, resides on chromosome 11. The central dogma of molecular biology – the flow of genetic information from DNA to RNA to protein – provides the framework for understanding how this mutation manifests as disease.
DNA: The Blueprint of Life
Deoxyribonucleic acid (DNA) is the fundamental hereditary material, a double-stranded helix composed of nucleotides. Each nucleotide consists of a deoxyribose sugar, a phosphate group, and one of four nitrogenous bases: adenine (A), guanine (G), cytosine (C), and thymine (T). The specific sequence of these bases along the DNA molecule constitutes the genetic code. The HBB gene, like all genes, contains the precise sequence of nucleotides necessary to encode the beta-globin subunit of hemoglobin.
Transcription: DNA to RNA
The first step in protein synthesis is transcription, where the DNA sequence of the HBB gene is transcribed into a messenger RNA (mRNA) molecule. This process involves the enzyme RNA polymerase, which binds to the gene's promoter region and unwinds the DNA double helix. RNA polymerase then synthesizes a complementary RNA molecule using the DNA strand as a template. In this RNA molecule, uracil (U) replaces thymine (T). The resulting mRNA molecule carries the genetic information from the nucleus to the ribosomes in the cytoplasm, the sites of protein synthesis. Mutations in the HBB gene, such as the single nucleotide substitution responsible for SCD, are directly transcribed into the mRNA.
Translation: RNA to Protein
Translation is the process where the mRNA sequence is translated into a polypeptide chain, the precursor to a functional protein. This occurs at the ribosomes, complex molecular machines that read the mRNA sequence in codons (three-nucleotide units). Each codon specifies a particular amino acid. Transfer RNA (tRNA) molecules, each carrying a specific amino acid, recognize and bind to their corresponding codons on the mRNA. The ribosome facilitates the formation of peptide bonds between the amino acids, building the polypeptide chain.
In the case of HBB, the mRNA sequence dictates the amino acid sequence of the beta-globin subunit. The specific mutation in SCD replaces a single adenine (A) nucleotide with a thymine (T), leading to a change in a single codon. This single nucleotide polymorphism (SNP) results in the substitution of glutamic acid (hydrophilic) with valine (hydrophobic) at the sixth position of the beta-globin chain. This seemingly minor change has profound consequences for the protein's structure and function.
The Impact of the Sickle Cell Mutation on Hemoglobin Structure and Function
The substitution of valine for glutamic acid in the beta-globin chain alters the overall structure of the hemoglobin molecule. Glutamic acid, with its charged side chain, is hydrophilic (water-loving), whereas valine, with its nonpolar side chain, is hydrophobic (water-fearing). This substitution introduces a hydrophobic patch on the surface of the hemoglobin molecule. Under low oxygen conditions, these hydrophobic patches on multiple hemoglobin molecules aggregate, causing the hemoglobin molecules to polymerize and form long, rigid fibers.
These fibers distort the shape of the red blood cells from their normal biconcave disc shape into a characteristic sickle or crescent shape. Sickled red blood cells are less flexible and more prone to clogging blood vessels, leading to vaso-occlusive crises – a hallmark of SCD. The rigid sickle cells also have a shorter lifespan, leading to hemolytic anemia, a condition characterized by a deficiency of red blood cells.
Consequences of Sickle Cell Hemoglobin:
- Vaso-occlusive crises: Blockage of blood vessels due to the rigid, sickle-shaped red blood cells, leading to pain, organ damage, and stroke.
- Hemolytic anemia: Premature destruction of red blood cells, resulting in a shortage of oxygen-carrying cells and anemia.
- Acute chest syndrome: A life-threatening complication involving lung inflammation and infection.
- Chronic organ damage: Repeated vaso-occlusive crises can lead to damage to various organs, including the kidneys, spleen, liver, and brain.
- Increased susceptibility to infections: The compromised spleen function in SCD patients increases their vulnerability to infections.
Diagnosis and Treatment of Sickle Cell Disease
Diagnosis of SCD typically involves a combination of blood tests, including hemoglobin electrophoresis and genetic testing. Hemoglobin electrophoresis separates different types of hemoglobin, allowing for the identification of sickle hemoglobin (HbS). Genetic testing confirms the presence of the HBB gene mutation. Early diagnosis is crucial for implementing timely interventions and improving the quality of life for individuals with SCD.
Treatment for SCD focuses on managing symptoms and preventing complications. Hydroxurea, a medication that increases the production of fetal hemoglobin (HbF), a form of hemoglobin resistant to sickling, is often used to reduce the frequency of vaso-occlusive crises. Other treatments include blood transfusions, pain management, and antibiotics to combat infections. Bone marrow transplantation, while a potentially curative treatment, is only feasible in a limited number of cases. Gene therapy, a promising area of research, offers the potential for a more widespread and effective cure for SCD. However, the complexity of the human genome means it's crucial for gene therapies to be tailored and well-tested.
Future Directions: Research and Gene Therapy
Research into SCD continues to advance our understanding of the disease mechanisms and explore new therapeutic avenues. Gene therapy, in particular, has emerged as a potential game-changer. This approach aims to correct the underlying genetic defect responsible for SCD by either modifying the existing HBB gene or introducing a functional copy of the gene into affected cells. Several gene therapy approaches are under development, showing promise in clinical trials, offering hope for a future where SCD is no longer a life-limiting condition. Further research is essential to refine gene editing technology, address potential safety concerns, and ensure equitable access to these innovative therapies. This includes researching the long-term effects of gene therapies and ensuring patient selection criteria are accurate and comprehensive.
The Broader Impact of Studying Sickle Cell Disease
The study of SCD has far-reaching implications beyond the disease itself. It has significantly advanced our understanding of protein structure-function relationships, the intricacies of gene regulation, and the power of genetic mutations to alter cellular processes. The lessons learned from SCD research have informed the development of therapeutic strategies for other genetic disorders and contributed to broader advancements in molecular biology and medicine. Understanding the complexities of SCD serves as a powerful reminder of the intricate relationship between genes, proteins, and human health. The ongoing research and development efforts for SCD contribute not just to improving the lives of those affected but also to the advancement of medical science as a whole, paving the way for effective treatments and cures for a wider range of genetic diseases. Further study of this disease will improve our understanding of the impact of genetic mutations on health, offering valuable insights for a broader understanding of human genetics and disease.
Latest Posts
Latest Posts
-
What Is Another Name For Line Qr
Apr 22, 2025
-
As The Play Opens What Disaster Has Befallen Thebes
Apr 22, 2025
-
An Ideal Habitat With Unlimited Resources Is Associated With
Apr 22, 2025
-
Based On The Bloomberg Eco Calendar If On 5 23
Apr 22, 2025
-
A Food Handlers Prescription Medicine Must Be Stored
Apr 22, 2025
Related Post
Thank you for visiting our website which covers about Investigation Dna Proteins And Sickle Cell . We hope the information provided has been useful to you. Feel free to contact us if you have any questions or need further assistance. See you next time and don't miss to bookmark.