Iron Undergoes An Allotropic Transformation At 912
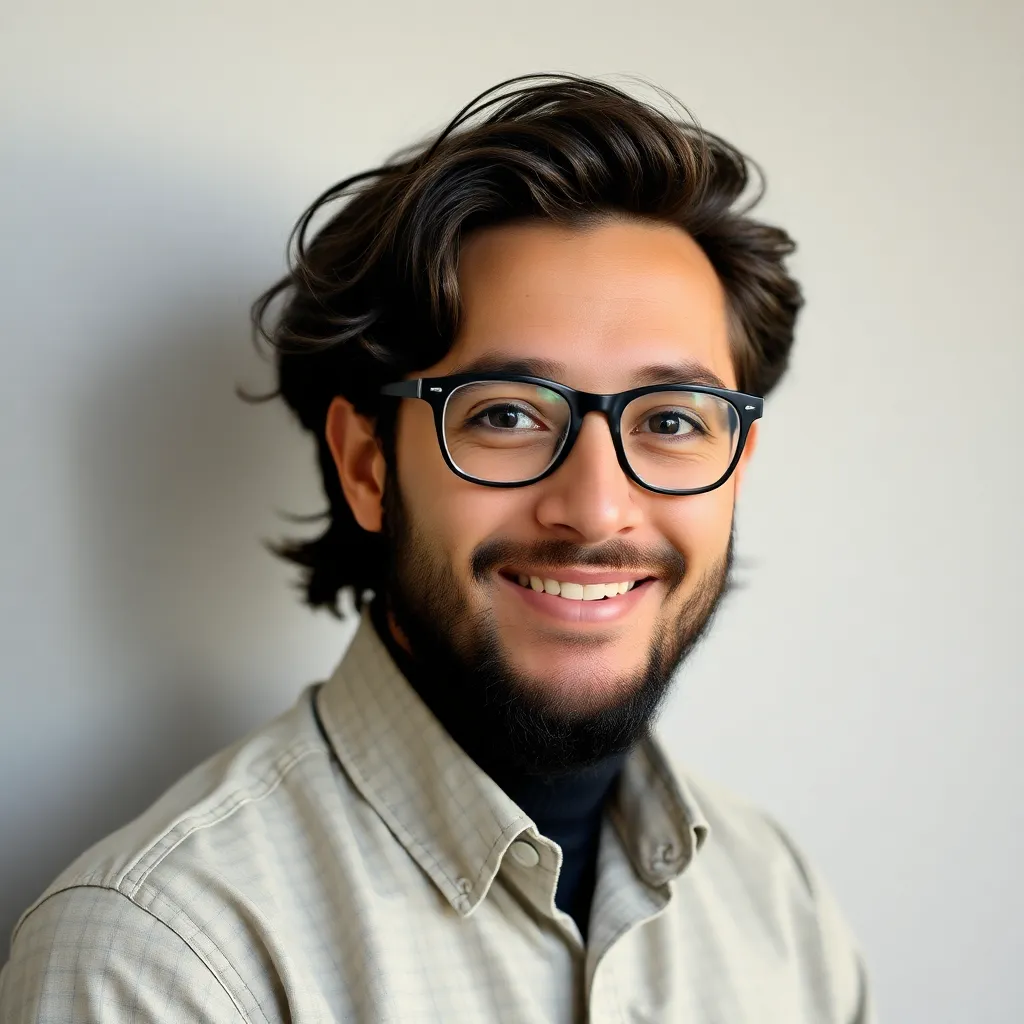
Onlines
May 08, 2025 · 6 min read
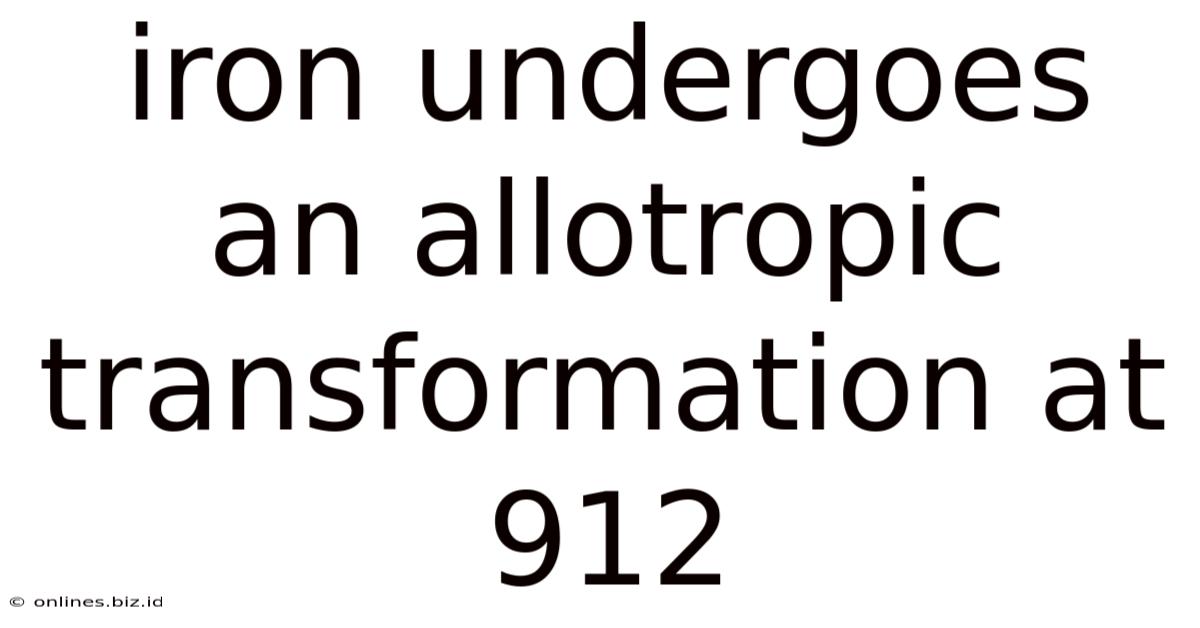
Table of Contents
Iron Undergoes an Allotropic Transformation at 912°C: A Deep Dive into its Crystalline Structure and Properties
Iron, a ubiquitous element fundamental to our technological civilization, exhibits fascinating behavior regarding its crystalline structure. One of the most significant aspects of its material properties is its allotropic transformation at approximately 912°C (1674°F). This transformation, a change in crystalline structure without a change in chemical composition, drastically impacts iron's properties and influences its use in diverse applications. Understanding this transformation is crucial for anyone working with iron or its alloys, from material scientists and engineers to metallurgists and blacksmiths.
Understanding Allotropy
Before delving into the specifics of iron's transformation, let's establish a foundational understanding of allotropy. Allotropy, also known as allotropism, refers to the ability of an element to exist in two or more different forms, known as allotropes, in the same physical state. These different forms possess distinct physical properties, such as crystal structure, density, hardness, and magnetic properties, owing to the arrangement of atoms within the crystal lattice. The conditions under which a specific allotrope is stable, such as temperature and pressure, determine which form will be present.
Iron's Allotropes: A Structural Overview
Iron exhibits three primary allotropes at atmospheric pressure, each characterized by a unique crystal structure:
Alpha-Iron (α-Fe)
- Temperature range: Below 912°C
- Crystal structure: Body-Centered Cubic (BCC)
- Magnetic properties: Ferromagnetic below 770°C (Curie temperature), becoming paramagnetic above this temperature. This transition is crucial in understanding the behavior of iron-based magnets.
- Properties: Relatively soft, ductile, and possesses good weldability. Its BCC structure contributes to its relatively low carbon solubility.
Gamma-Iron (γ-Fe)
- Temperature range: 912°C to 1394°C
- Crystal structure: Face-Centered Cubic (FCC)
- Magnetic properties: Paramagnetic
- Properties: Higher carbon solubility compared to α-Fe, leading to its importance in steelmaking. Its FCC structure results in greater ductility and higher stacking fault energy.
Delta-Iron (δ-Fe)
- Temperature range: 1394°C to 1538°C (melting point)
- Crystal structure: Body-Centered Cubic (BCC)
- Magnetic properties: Paramagnetic
- Properties: Similar to α-Fe but with slightly different properties due to the higher temperature. The higher temperature influences the diffusion rate of carbon and other alloying elements, impacting its behavior during processing.
The 912°C Transformation: A Critical Point
The allotropic transformation at 912°C marks a significant shift in iron's properties. This transition involves a change from the BCC structure of α-iron to the FCC structure of γ-iron. This structural change isn't instantaneous; it involves a complex rearrangement of atoms within the crystal lattice. The transformation is typically accompanied by a change in volume, although it's relatively small.
Understanding the mechanics of this transformation requires considering several factors:
- Nucleation: The process begins with the formation of small nuclei of the new γ-iron phase within the existing α-iron structure. These nuclei act as seeds for the growth of the new phase.
- Growth: The γ-iron nuclei grow by the diffusion of iron atoms from the α-iron to the γ-iron phase. This process is temperature-dependent, with higher temperatures promoting faster diffusion.
- Interface migration: The boundary between the α-iron and γ-iron phases moves as the transformation progresses. The speed of this migration is influenced by factors like temperature, impurities, and grain size.
The transformation at 912°C is a first-order transformation, meaning there's a significant change in enthalpy and volume during the transition. This change is directly related to the differences in atomic packing efficiency between the BCC and FCC structures. The FCC structure allows for more efficient packing of atoms, resulting in a slightly higher density.
Impact on Material Properties
The 912°C allotropic transformation profoundly impacts several key properties of iron:
- Hardness and Strength: The transformation influences the mechanical strength and hardness of iron. The FCC structure of γ-iron generally results in higher ductility and lower strength compared to the BCC structure of α-iron. This is related to the ease of dislocation motion in FCC crystals.
- Ductility and Malleability: γ-iron tends to be more ductile and malleable than α-iron, making it easier to shape and form. This is due to the different slip systems available in the FCC crystal structure.
- Magnetic Properties: The transition from ferromagnetic α-iron to paramagnetic γ-iron above the Curie temperature (770°C) significantly alters its magnetic behavior.
- Solubility of Carbon: The higher solubility of carbon in γ-iron is essential in steelmaking. The ability to dissolve substantial amounts of carbon within the austenite (γ-iron) phase at high temperatures allows for controlled cooling processes to produce various steel microstructures with tailored properties. This impacts the heat treatment processes employed to enhance the properties of steel.
Practical Applications and Significance
The understanding of iron's allotropic transformation is paramount across numerous industries and applications. Its significance is particularly evident in:
- Steelmaking: The controlled transformation between the different allotropes of iron is fundamental to the heat treatments employed in steel manufacturing. By carefully controlling the cooling rate, metallurgists can manipulate the microstructural features (like the formation of pearlite, martensite, or bainite) and thus tailor the mechanical properties of the resulting steel for specific applications, ranging from structural steel to high-strength alloys.
- Welding: The transformation plays a critical role in welding processes. The heating and cooling cycles involved in welding can lead to changes in the microstructure and hence the properties of the welded joint. Understanding the transformation is crucial to prevent defects and ensure the integrity of the weld.
- Casting: The phase transformation impacts the solidification behavior of iron and its alloys during casting processes. Controlling the cooling rate influences the grain size and microstructure, which ultimately affects the final properties of the casting.
- Heat treatment: Various heat treatment processes, including annealing, normalizing, and quenching, rely on the precise control of the allotropic transformations to achieve desired material properties. The interplay between temperature, time, and cooling rate is precisely manipulated to obtain specific microstructures.
Further Research and Exploration
The study of iron's allotropic transformations continues to be a rich area of research. Scientists are constantly exploring the finer details of the transformation mechanisms, investigating the influence of alloying elements, and developing advanced characterization techniques to gain a deeper understanding. Further research could focus on:
- Influence of alloying elements: Investigating how alloying elements affect the transformation temperature, kinetics, and resulting microstructure. Various alloying elements are added to iron to modify its properties and create specific steel grades with tailored characteristics.
- Advanced characterization techniques: Employing advanced techniques such as in-situ microscopy to directly observe the transformation process at the atomic level.
- Predictive modeling: Developing accurate predictive models that can simulate the transformation behavior under various conditions.
Conclusion
The allotropic transformation of iron at 912°C is a crucial phenomenon that profoundly influences its properties and applications. This transformation, involving a change from the BCC structure of α-iron to the FCC structure of γ-iron, affects various material properties, including hardness, ductility, and carbon solubility. Understanding this transformation is critical across numerous industries, particularly in steelmaking, welding, and casting. Ongoing research continues to unravel the complexities of this transformation, leading to improvements in materials processing and the development of novel iron-based materials with enhanced properties. The fascinating interplay between crystal structure and material properties makes iron a continuously fascinating subject of study and innovation.
Latest Posts
Latest Posts
-
C Span Classroom The Powers Of Congress Handout Answers
May 08, 2025
-
Documents Which Reflect On The Character Performance
May 08, 2025
-
A Student Studied The Clock Reaction Described In This Experiment
May 08, 2025
-
Legalizing All Forms Of Illegal Activities
May 08, 2025
-
Drag The Appropriate Items Into Their Respective Bins
May 08, 2025
Related Post
Thank you for visiting our website which covers about Iron Undergoes An Allotropic Transformation At 912 . We hope the information provided has been useful to you. Feel free to contact us if you have any questions or need further assistance. See you next time and don't miss to bookmark.