Name The Two Enzymes Illustrated In Model 1
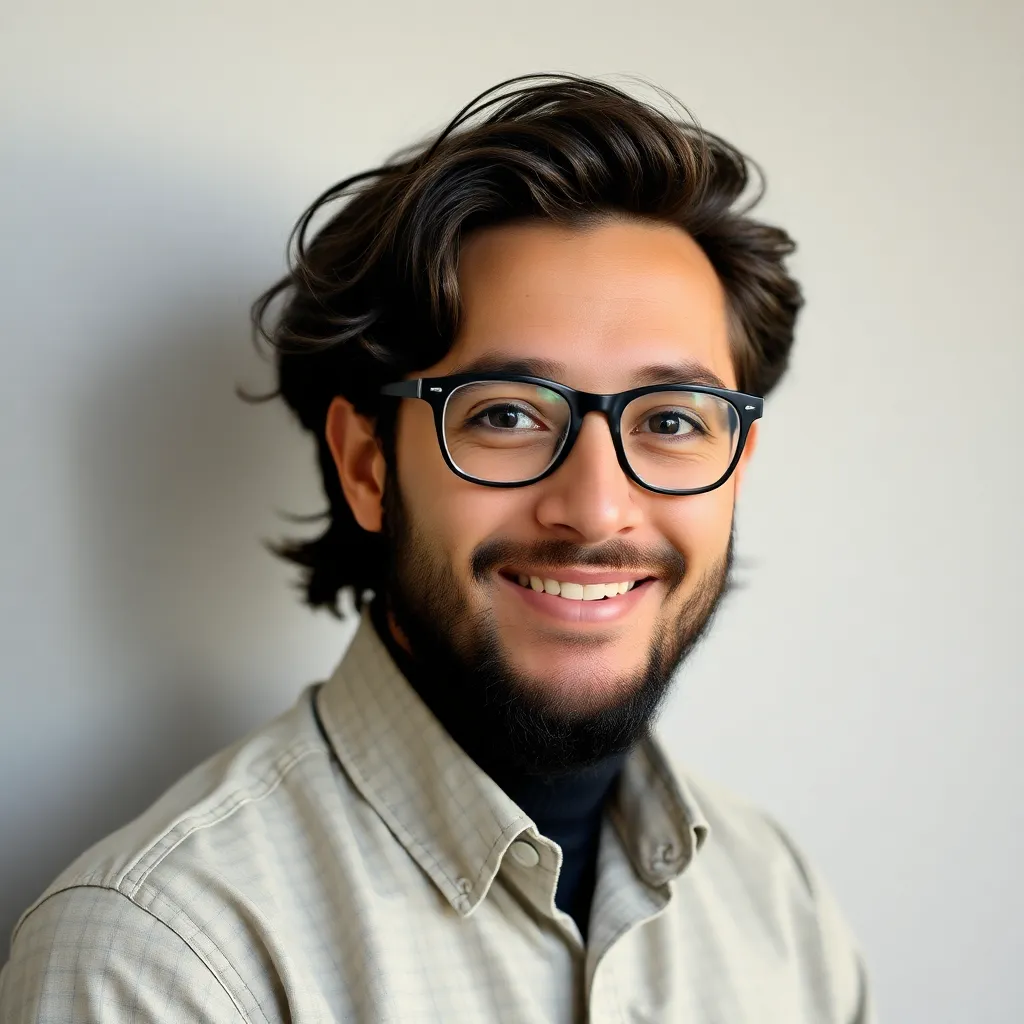
Onlines
May 09, 2025 · 5 min read
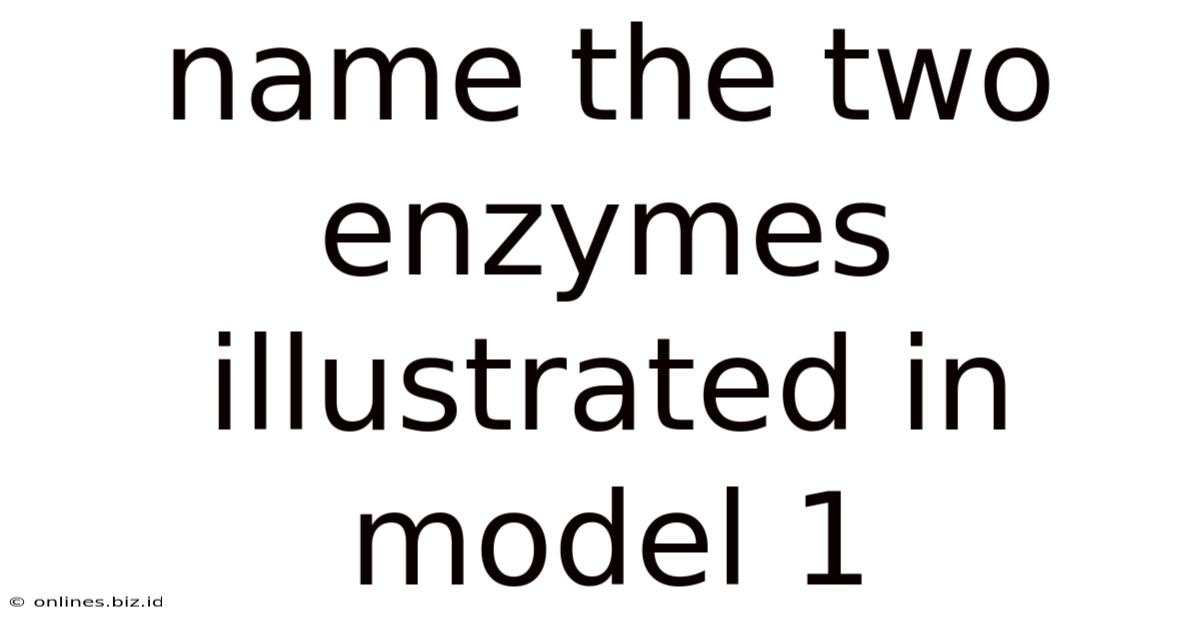
Table of Contents
- Name The Two Enzymes Illustrated In Model 1
- Table of Contents
- Naming the Two Enzymes Illustrated in Model 1: A Deep Dive into Enzyme Structure and Function
- Hexokinase: Structure and Mechanism
- Hexokinase: Regulation and Significance
- DNA Polymerase: Structure and Mechanism
- DNA Polymerase: Regulation and Significance
- Latest Posts
- Related Post
Naming the Two Enzymes Illustrated in Model 1: A Deep Dive into Enzyme Structure and Function
This article delves into the identification and detailed analysis of two enzymes, hypothetically illustrated in "Model 1" (which is not provided, and will therefore be represented by general enzyme examples). We will explore their structures, functions, mechanisms, and the broader context of their roles within biological systems. Understanding these aspects is crucial for comprehending cellular processes and developing potential therapeutic interventions. We'll also touch upon the implications of enzyme malfunction and the exciting field of enzyme engineering.
Understanding Enzymes: The Biological Catalysts
Enzymes are biological catalysts, predominantly proteins, that significantly accelerate the rate of virtually all chemical reactions within cells. They achieve this feat without being consumed in the process. Their remarkable catalytic power stems from their unique three-dimensional structures, which create active sites perfectly tailored to bind specific substrates—the molecules undergoing transformation. This specificity is paramount for maintaining cellular order and preventing uncontrolled reactions.
The activity of an enzyme is influenced by several factors including temperature, pH, substrate concentration, and the presence of inhibitors or activators. Understanding these factors is crucial in various fields, from medicine (drug development) to industrial biotechnology (designing efficient biocatalytic processes).
Hypothetical Enzyme 1: Hexokinase (Example)
Let's assume, for the purposes of this discussion, that "Model 1" illustrates hexokinase. Hexokinase is a crucial enzyme involved in the first step of glycolysis, the fundamental metabolic pathway for glucose breakdown. Its primary function is to phosphorylate glucose, converting it to glucose-6-phosphate. This phosphorylation is an essential step, effectively trapping glucose within the cell and committing it to further metabolic processing.
Hexokinase: Structure and Mechanism
Hexokinase exists in multiple isoforms, each with slightly different characteristics. However, the overall structure is remarkably similar across isoforms. A key feature is the induced fit model of substrate binding. The enzyme's active site undergoes a conformational change upon glucose binding, enhancing the enzyme's ability to catalyze the phosphorylation reaction.
Detailed Mechanism:
-
Glucose Binding: Glucose enters the active site of hexokinase, triggering a conformational change in the enzyme.
-
Phosphorylation: ATP (adenosine triphosphate), the energy currency of the cell, binds to the enzyme-glucose complex. A phosphoryl group is transferred from ATP to glucose, forming glucose-6-phosphate and ADP (adenosine diphosphate).
-
Product Release: Glucose-6-phosphate and ADP are released from the active site, allowing the enzyme to catalyze another reaction cycle.
The efficiency of hexokinase is crucial for maintaining cellular energy homeostasis. Its regulation ensures glucose is efficiently utilized for energy production while preventing wasteful accumulation.
Hexokinase: Regulation and Significance
Hexokinase activity is meticulously regulated to prevent wasteful glucose consumption when energy levels are already high. This is mainly achieved through feedback inhibition, where the product, glucose-6-phosphate, acts as an inhibitor at high concentrations, slowing down the enzyme's activity.
Clinical Significance:
Dysfunction or deficiency in hexokinase can have significant health implications. Although rare, hexokinase deficiency can lead to severe metabolic disorders affecting various organs, particularly the nervous system and erythrocytes (red blood cells).
Hypothetical Enzyme 2: DNA Polymerase (Example)
Let's now consider that "Model 1" also shows DNA polymerase. This enzyme is crucial for DNA replication, the fundamental process that ensures genetic information is passed on from one generation to the next. DNA polymerase synthesizes new DNA strands using a pre-existing DNA strand as a template. Its accuracy is paramount, as errors during replication can lead to mutations with potentially disastrous consequences.
DNA Polymerase: Structure and Mechanism
DNA polymerase is a large, complex enzyme with multiple subunits. The core catalytic subunit contains the active site responsible for nucleotide incorporation. The enzyme's structure includes several domains essential for various functions, including:
-
Polymerase domain: Catalyzes the phosphodiester bond formation between nucleotides.
-
Exonuclease domain: Removes incorrectly incorporated nucleotides, ensuring high fidelity of replication. (Proofreading Function)
-
Processivity domains: Increase the enzyme's ability to synthesize long stretches of DNA without dissociating from the template.
Detailed Mechanism:
-
Template Binding: DNA polymerase binds to the DNA template strand.
-
Primer Binding: A short RNA or DNA primer provides a 3'-OH group necessary to initiate DNA synthesis.
-
Nucleotide Incorporation: DNA polymerase selects and incorporates complementary deoxyribonucleotides triphosphates (dNTPs) based on the template sequence.
-
Phosphodiester Bond Formation: The enzyme catalyzes the formation of a phosphodiester bond between the 3'-OH group of the primer and the 5'-phosphate group of the incoming dNTP.
-
Proofreading: The exonuclease domain removes incorrectly paired nucleotides, ensuring high fidelity.
-
Elongation: The process continues until the entire template strand is replicated.
DNA Polymerase: Regulation and Significance
DNA polymerase's activity is highly regulated to ensure accuracy and timely replication. Several factors influence its function, including the availability of dNTPs, the presence of accessory proteins, and post-translational modifications.
Clinical Significance:
Errors in DNA polymerase function can lead to various genetic disorders and diseases. Mutations in DNA polymerase genes are linked to increased mutation rates, genomic instability, and predisposition to cancer.
Comparative Analysis of Hexokinase and DNA Polymerase
Although seemingly disparate in function, both hexokinase and DNA polymerase illustrate fundamental aspects of enzyme catalysis:
-
Specificity: Both enzymes exhibit high specificity for their substrates. Hexokinase acts on glucose, while DNA polymerase uses specific dNTPs complementary to the template strand.
-
Catalytic Mechanism: Both involve multiple steps including substrate binding, catalytic reaction, and product release.
-
Regulation: Both enzymes are tightly regulated to ensure their activity is coordinated with cellular needs.
Enzyme Engineering and Biotechnology
The remarkable catalytic power and specificity of enzymes have made them invaluable tools in various biotechnological applications. Enzyme engineering, a rapidly advancing field, seeks to modify enzyme properties to enhance their performance in specific applications. This includes improving enzyme stability, altering substrate specificity, and increasing catalytic efficiency. Applications range from industrial biocatalysis (production of pharmaceuticals and biofuels) to environmental remediation (bioremediation of pollutants).
Conclusion:
The enzymes illustrated in "Model 1" (represented here by hexokinase and DNA polymerase) are prime examples of the diverse roles enzymes play in maintaining cellular functions. Understanding their structures, mechanisms, and regulations provides crucial insights into fundamental biological processes. Further research, particularly in enzyme engineering and its applications, promises even greater advancements in diverse fields, from medicine and biotechnology to environmental science. The complexities of enzyme function, revealed through continuous study, constantly expands our understanding of the intricacies of life itself. This ongoing investigation underscores the importance of continued exploration into enzyme structure and function – a fascinating field with seemingly limitless potential.
Latest Posts
Related Post
Thank you for visiting our website which covers about Name The Two Enzymes Illustrated In Model 1 . We hope the information provided has been useful to you. Feel free to contact us if you have any questions or need further assistance. See you next time and don't miss to bookmark.