Neuron Anatomy And Physiology Review Sheet Exercise 13
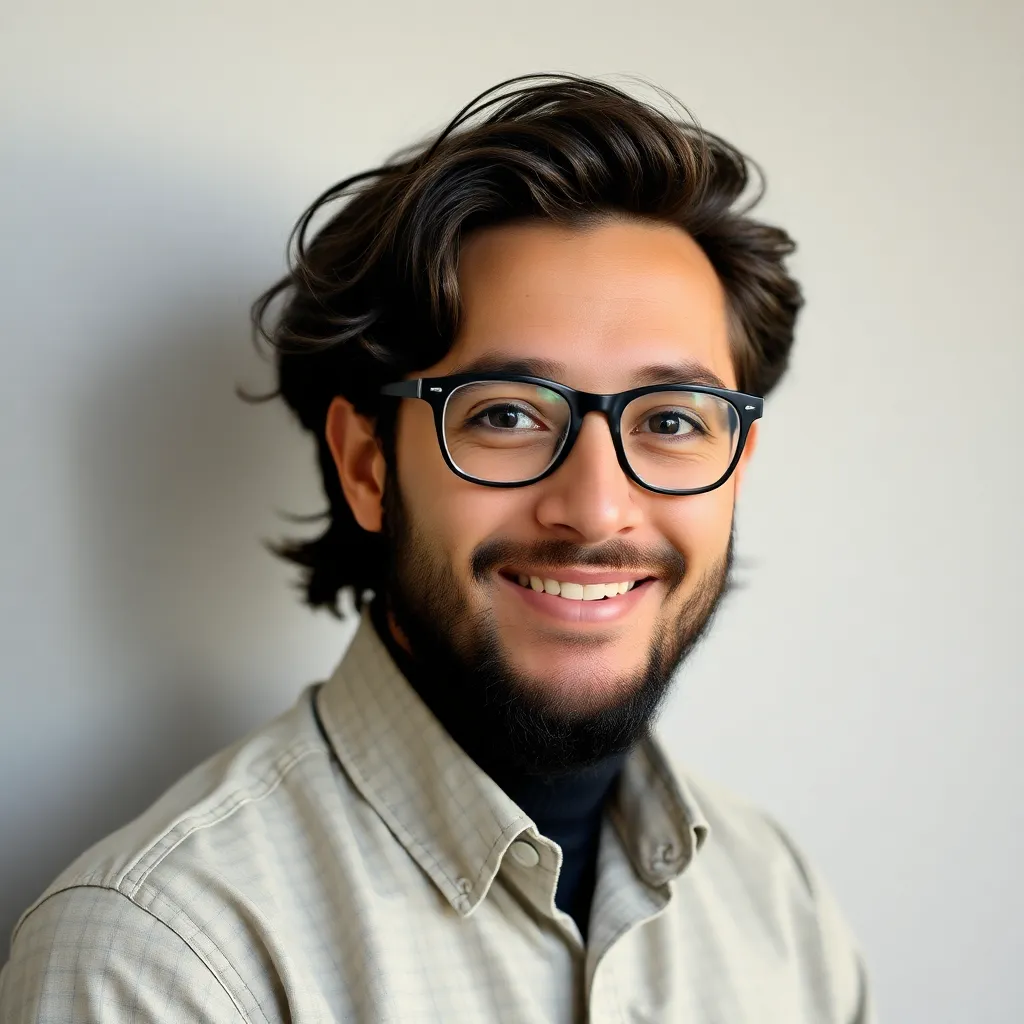
Onlines
Apr 06, 2025 · 8 min read
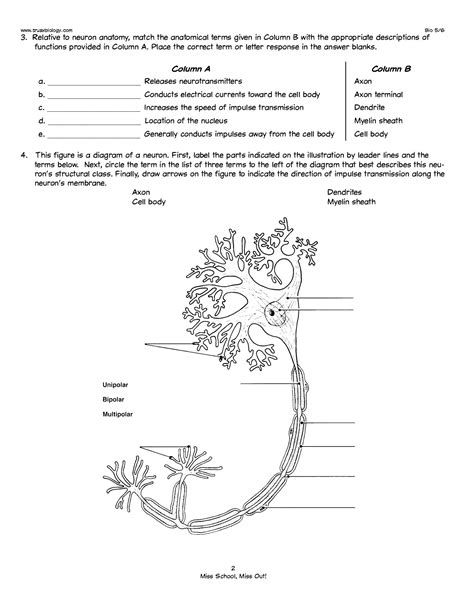
Table of Contents
Neuron Anatomy and Physiology Review Sheet: Exercise 13
This comprehensive review sheet covers the anatomy and physiology of neurons, providing a detailed look at their structure and function. We'll delve into the intricate details, making this an invaluable resource for students and anyone seeking a thorough understanding of these fundamental units of the nervous system. This exercise will reinforce your learning through various methods, including definitions, diagrams, and application-based questions.
I. Neuron Structure: A Detailed Look
Neurons, the basic functional units of the nervous system, are highly specialized cells responsible for receiving, processing, and transmitting information. Their unique structure is intimately tied to their function. Let's examine the key components:
A. Soma (Cell Body): The Neuron's Control Center
The soma, or cell body, is the neuron's central hub. It contains the nucleus, which houses the cell's genetic material (DNA), and other essential organelles like mitochondria (for energy production), ribosomes (for protein synthesis), and the endoplasmic reticulum (for protein folding and transport). The soma integrates incoming signals from dendrites and initiates outgoing signals down the axon.
Key Features of the Soma:
- Nissl bodies: Clusters of rough endoplasmic reticulum responsible for protein synthesis, crucial for neuronal function and maintenance.
- Neurofilaments: Provide structural support to the soma and maintain its shape.
- Golgi apparatus: Modifies and packages proteins for transport within the neuron or secretion.
B. Dendrites: Receiving Information
Dendrites are branching extensions of the soma that receive signals from other neurons. Their extensive branching pattern significantly increases the surface area available for synaptic input. These signals, in the form of neurotransmitters, bind to receptors on the dendritic membrane, triggering electrical changes that propagate towards the soma.
Characteristics of Dendrites:
- Dendritic spines: Small protrusions along the dendrites that increase the surface area for synaptic connections, influencing synaptic plasticity and learning.
- Receptor proteins: Specialized proteins on the dendritic membrane that bind to neurotransmitters, initiating signal transduction.
C. Axon: Transmitting Information
The axon is a long, slender projection extending from the soma, responsible for transmitting signals to other neurons, muscles, or glands. The axon's structure is crucial for efficient signal conduction.
Key Aspects of the Axon:
- Axon hillock: The region where the axon originates from the soma; this is the site of action potential initiation.
- Axon terminals (terminal boutons): The branched endings of the axon that form synapses with other neurons or target cells.
- Myelin sheath: A fatty insulating layer surrounding many axons, significantly increasing the speed of signal conduction (saltatory conduction). The myelin sheath is formed by oligodendrocytes in the central nervous system (CNS) and Schwann cells in the peripheral nervous system (PNS).
- Nodes of Ranvier: Gaps in the myelin sheath where the axon membrane is exposed; action potentials jump between these nodes, accelerating transmission.
II. Neuron Physiology: Signal Transmission
Neurons communicate via electrochemical signals. This involves the generation and propagation of action potentials and the release of neurotransmitters at synapses.
A. Membrane Potential: The Electrical Gradient
The neuronal membrane maintains an electrical potential difference across it, known as the membrane potential. This difference arises from the unequal distribution of ions (primarily sodium (Na+), potassium (K+), chloride (Cl-), and calcium (Ca2+)) across the membrane. The resting membrane potential is typically around -70 mV, meaning the inside of the neuron is more negative than the outside.
B. Action Potential: The All-or-None Signal
An action potential is a rapid, transient depolarization of the neuronal membrane. It's an all-or-none event: either it occurs completely or not at all. The process involves:
- Depolarization: Sodium channels open, causing a rapid influx of Na+ ions, making the inside of the neuron more positive.
- Repolarization: Potassium channels open, causing an efflux of K+ ions, restoring the negative membrane potential.
- Hyperpolarization: A brief period where the membrane potential becomes more negative than the resting potential before returning to normal.
C. Synaptic Transmission: Communication Between Neurons
Synapses are specialized junctions between neurons where communication occurs. The process involves:
- Neurotransmitter release: When an action potential reaches the axon terminal, it triggers the release of neurotransmitters into the synaptic cleft (the gap between neurons).
- Neurotransmitter binding: Neurotransmitters diffuse across the cleft and bind to receptors on the postsynaptic neuron's membrane.
- Postsynaptic potential: The binding of neurotransmitters induces a change in the postsynaptic neuron's membrane potential, either excitatory postsynaptic potential (EPSP) – causing depolarization, or inhibitory postsynaptic potential (IPSP) – causing hyperpolarization.
- Signal integration: The soma integrates the combined effects of EPSPs and IPSPs to determine whether or not an action potential will be generated.
D. Neurotransmitters: Chemical Messengers
Numerous neurotransmitters exist, each with specific effects on postsynaptic neurons. Some examples include:
- Acetylcholine: Involved in muscle contraction, memory, and learning.
- Dopamine: Involved in reward, motivation, and movement.
- Serotonin: Involved in mood regulation, sleep, and appetite.
- GABA (gamma-aminobutyric acid): The primary inhibitory neurotransmitter in the CNS.
- Glutamate: The primary excitatory neurotransmitter in the CNS.
III. Types of Neurons: Functional Classification
Neurons are classified based on their function and structure.
A. Sensory Neurons (Afferent Neurons):
These neurons transmit information from sensory receptors to the central nervous system. They detect stimuli such as light, sound, touch, temperature, and pain. Their cell bodies are typically located in ganglia outside the CNS.
B. Motor Neurons (Efferent Neurons):
These neurons transmit signals from the CNS to muscles or glands, causing them to contract or secrete substances. Their cell bodies are located within the CNS.
C. Interneurons:
These neurons connect sensory and motor neurons within the CNS, facilitating complex information processing. They form the majority of neurons in the brain and spinal cord.
IV. Neuroglia: Support Cells of the Nervous System
Neuroglia, or glial cells, are non-neuronal cells that provide structural and functional support to neurons. They are crucial for maintaining neuronal health and function. Major types include:
- Astrocytes: Provide structural support, regulate blood flow, and maintain the blood-brain barrier.
- Oligodendrocytes (CNS) and Schwann cells (PNS): Form the myelin sheath around axons.
- Microglia: Act as immune cells of the CNS, removing debris and pathogens.
- Ependymal cells: Line the ventricles of the brain and the central canal of the spinal cord, producing cerebrospinal fluid.
V. Exercise Questions: Testing Your Knowledge
To solidify your understanding of neuron anatomy and physiology, let's try some application-based questions.
1. Describe the process of saltatory conduction and explain its significance.
Answer: Saltatory conduction is the rapid propagation of action potentials along myelinated axons. The action potential "jumps" between the Nodes of Ranvier, the gaps in the myelin sheath. This jumping process is significantly faster than continuous conduction in unmyelinated axons. Its significance lies in its ability to greatly increase the speed of nerve impulse transmission, allowing for faster reflexes and more efficient information processing.
2. Explain the difference between an EPSP and an IPSP. How do these contribute to signal integration at the axon hillock?
Answer: An excitatory postsynaptic potential (EPSP) is a depolarization of the postsynaptic membrane, making it more likely to fire an action potential. An inhibitory postsynaptic potential (IPSP) is a hyperpolarization of the postsynaptic membrane, making it less likely to fire an action potential. At the axon hillock, the site of action potential initiation, the neuron integrates the summed effects of all EPSPs and IPSPs. If the net effect is a sufficient depolarization to reach the threshold potential, an action potential is generated. If the net effect is insufficient, no action potential occurs.
3. Describe the role of myelin in the conduction of action potentials. What happens in diseases that demyelinate axons (e.g., multiple sclerosis)?
Answer: Myelin acts as an insulator around axons, preventing ion leakage and allowing action potentials to jump between the Nodes of Ranvier (saltatory conduction). This significantly increases the speed of nerve impulse transmission. In demyelinating diseases like multiple sclerosis, the myelin sheath is damaged or destroyed. This disrupts saltatory conduction, slowing down or blocking nerve impulse transmission. This leads to a wide range of neurological symptoms depending on which axons are affected.
4. What are the different types of neuroglia and what are their primary functions?
Answer: The primary types of neuroglia are: Astrocytes (structural support, blood-brain barrier, nutrient transport), Oligodendrocytes (CNS myelin formation), Schwann cells (PNS myelin formation), Microglia (immune function), and Ependymal cells (cerebrospinal fluid production). Each plays a vital role in supporting neuron function and maintaining the health of the nervous system.
5. Explain the role of neurotransmitters in synaptic transmission. Give examples of excitatory and inhibitory neurotransmitters.
Answer: Neurotransmitters are chemical messengers released from axon terminals into the synaptic cleft. They bind to receptors on the postsynaptic membrane, causing either depolarization (excitatory postsynaptic potential, EPSP) or hyperpolarization (inhibitory postsynaptic potential, IPSP). Glutamate is a primary example of an excitatory neurotransmitter, while GABA is a primary example of an inhibitory neurotransmitter. The specific effect of a neurotransmitter depends on the type of receptor it binds to.
This comprehensive review sheet provides a strong foundation for understanding neuron anatomy and physiology. Remember to review these concepts thoroughly and use the practice questions to assess your understanding. Continued learning and application of this knowledge will further solidify your comprehension of this fascinating field.
Latest Posts
Latest Posts
-
The Combining Form That Means Pain Is
Apr 08, 2025
-
To Kill A Mockingbird Chapter 23 Summary
Apr 08, 2025
-
Summary Of Chapter 16 Catcher In The Rye
Apr 08, 2025
-
1984 Part 2 Chapter 2 Summary
Apr 08, 2025
-
The Projected Unit Sales Volume Of Branded
Apr 08, 2025
Related Post
Thank you for visiting our website which covers about Neuron Anatomy And Physiology Review Sheet Exercise 13 . We hope the information provided has been useful to you. Feel free to contact us if you have any questions or need further assistance. See you next time and don't miss to bookmark.