No Amino Acid Molecule By Itself
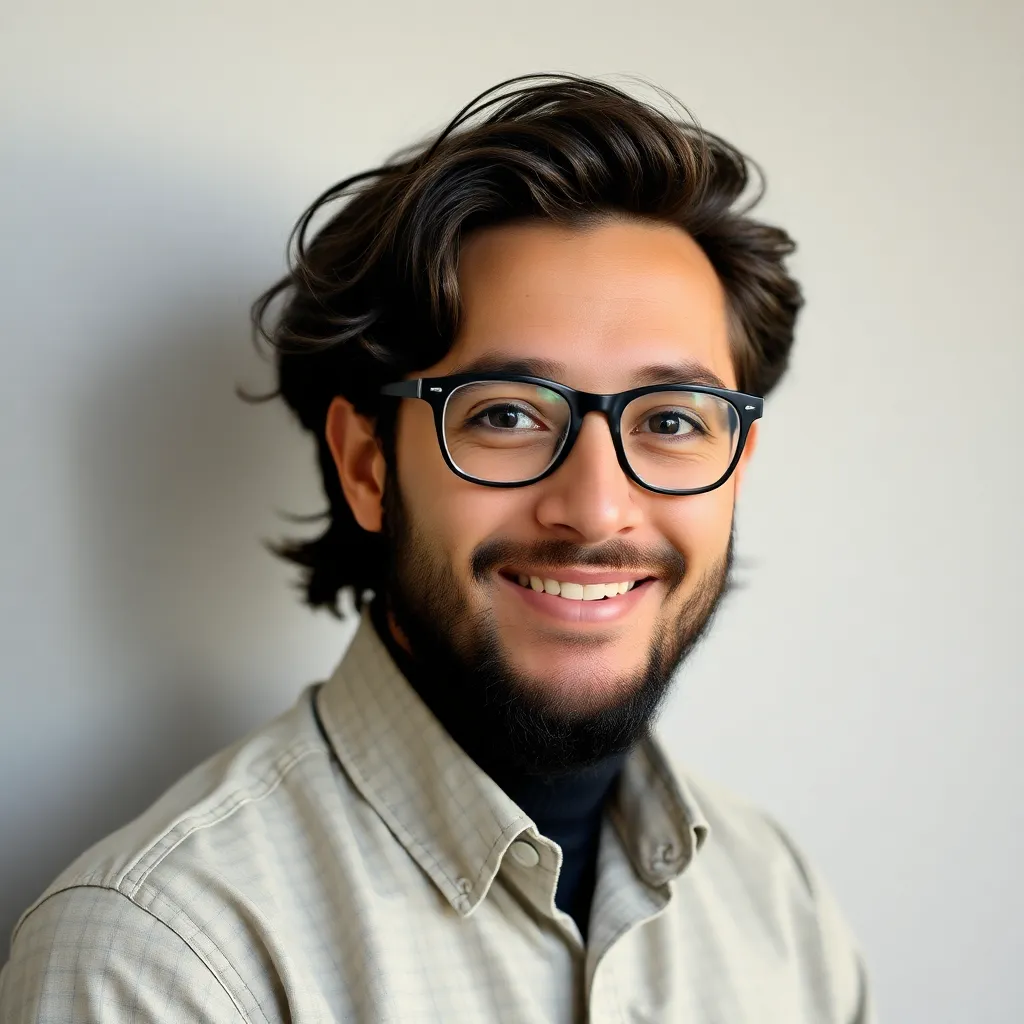
Onlines
May 12, 2025 · 6 min read
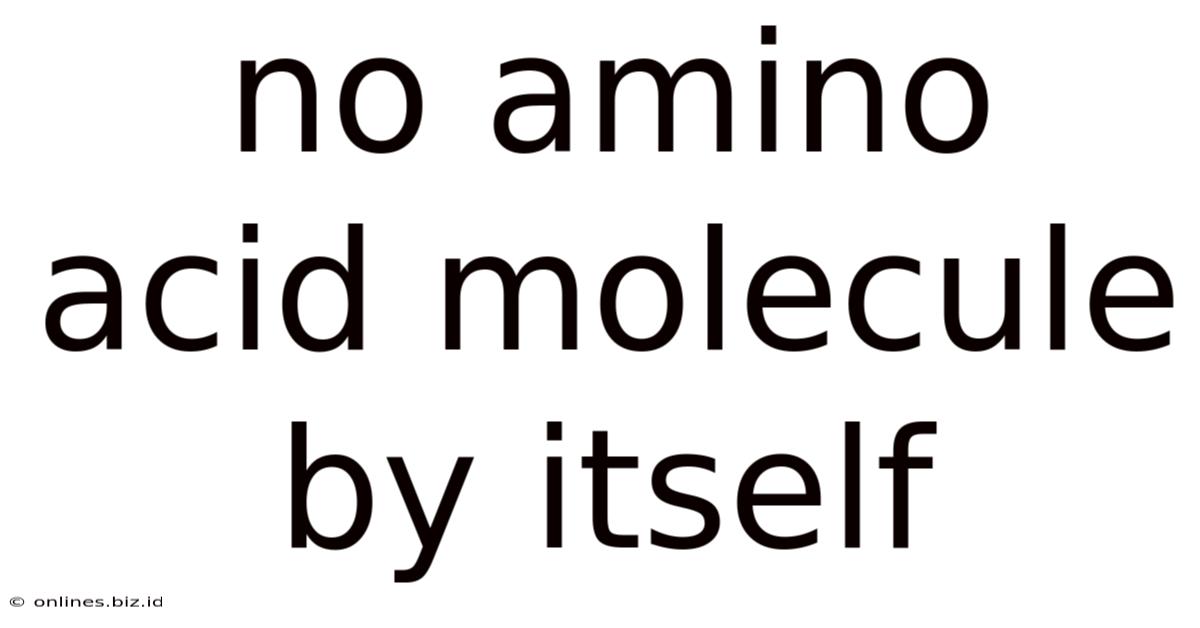
Table of Contents
No Amino Acid Molecule by Itself: Understanding the Importance of Peptide Chains and Protein Structure
Amino acids are the fundamental building blocks of proteins, but it's crucial to understand that no amino acid molecule functions independently in its isolated form. Their biological significance lies entirely in their ability to link together, forming chains and complex three-dimensional structures. This article delves deep into why single amino acids are biologically inactive and how their remarkable properties emerge only within the context of peptides and proteins.
The Lone Amino Acid: Biologically Inert
A single amino acid, existing in isolation, lacks the structural complexity and specific functionality necessary for biological activity. To understand this, let's consider the basic structure of an amino acid:
The Basic Structure and its Limitations
Every amino acid (except proline) possesses a central carbon atom (the α-carbon) bonded to four distinct groups:
- An amino group (-NH₂): This group is basic and can accept a proton.
- A carboxyl group (-COOH): This group is acidic and can donate a proton.
- A hydrogen atom (-H): A simple hydrogen atom.
- A side chain (R-group): This group varies significantly among the 20 standard amino acids and determines the unique properties of each.
While these functional groups possess chemical reactivity, the single amino acid molecule, isolated from others, cannot perform the myriad of biological tasks proteins carry out. It simply lacks the necessary structural framework and organized interaction sites.
The Power of Peptide Bonds: Linking Amino Acids
The biological activity of amino acids arises from their ability to form peptide bonds. This covalent bond links the carboxyl group of one amino acid to the amino group of another, releasing a water molecule in the process. This reaction is a dehydration synthesis. This linking creates a peptide, a chain of amino acids.
Peptide Chain Formation: A Dehydration Reaction
The formation of a peptide bond is a crucial step. It involves the removal of a water molecule and the formation of a strong amide linkage between the carbon of the carboxyl group and the nitrogen of the amino group. The peptide bond itself exhibits partial double-bond character due to resonance, making it relatively rigid and planar.
This rigidity is important for protein folding and function. It influences the angles between amino acids, affecting the overall conformation and properties of the resulting polypeptide chain.
The N-terminus and C-terminus: Directionality of the Chain
Peptide chains have directionality. One end has a free amino group (the N-terminus) and the other has a free carboxyl group (the C-terminus). This directionality is vital for protein synthesis and function, influencing how the chain folds and interacts with other molecules.
From Peptide to Protein: Emergence of Structure and Function
A simple peptide chain is still far from a fully functional protein. Proteins achieve their biological activities through intricate three-dimensional structures that arise from the interactions between their constituent amino acids. These levels of protein structure are crucial to understand:
Primary Structure: The Amino Acid Sequence
The primary structure is simply the linear sequence of amino acids in the polypeptide chain. This sequence is genetically encoded and dictates all subsequent levels of structure. Even a single amino acid change in the primary sequence can dramatically alter the protein's properties. This is seen in diseases like sickle cell anemia, caused by a single amino acid substitution in hemoglobin.
Secondary Structure: Local Folding Patterns
The primary structure folds into localized, repeating patterns, forming the secondary structure. These patterns are stabilized by hydrogen bonds between the peptide backbone atoms. Common secondary structures include:
- α-helices: A coiled structure stabilized by hydrogen bonds between every fourth amino acid.
- β-sheets: Extended polypeptide chains arranged side-by-side, stabilized by hydrogen bonds between adjacent strands.
- Loops and turns: Irregular regions that connect α-helices and β-sheets.
The specific secondary structures adopted by a protein depend on the sequence of amino acids in its primary structure.
Tertiary Structure: The 3D Conformation
The tertiary structure refers to the overall three-dimensional arrangement of a polypeptide chain. This structure is determined by interactions between the side chains (R-groups) of the amino acids. These interactions can be:
- Hydrophobic interactions: Nonpolar side chains cluster together in the protein's core, away from water.
- Hydrogen bonds: Polar side chains form hydrogen bonds with each other or with water molecules.
- Ionic bonds (salt bridges): Oppositely charged side chains attract each other.
- Disulfide bonds: Covalent bonds formed between cysteine residues.
The tertiary structure dictates the protein's overall shape and its biological activity. The precise folding is crucial for the protein to bind to its target molecules or substrates and perform its function.
Quaternary Structure: Multiple Polypeptide Chains
Some proteins consist of multiple polypeptide chains (subunits) arranged together, forming the quaternary structure. These subunits can be identical or different. Interactions between the subunits, similar to those in tertiary structure, stabilize the quaternary structure. Hemoglobin, for instance, has a quaternary structure composed of four subunits.
The Importance of Protein Folding and Chaperones
The folding of a polypeptide chain into its correct three-dimensional structure is a complex and crucial process. Mistakes in folding can lead to non-functional or even harmful proteins, contributing to diseases like Alzheimer's and Parkinson's.
Molecular chaperones are proteins that assist in the correct folding of other proteins. They prevent aggregation and misfolding, ensuring that proteins attain their functional conformations.
Diverse Roles of Proteins: A Consequence of Structure
The incredible diversity of protein functions stems directly from the vast possibilities in amino acid sequence and the resulting complexity of protein structures. Proteins perform a myriad of roles in living organisms, including:
- Enzymes: Catalyze biochemical reactions.
- Structural proteins: Provide support and shape to cells and tissues (e.g., collagen, keratin).
- Transport proteins: Carry molecules across cell membranes (e.g., hemoglobin).
- Hormones: Act as chemical messengers (e.g., insulin, growth hormone).
- Antibodies: Part of the immune system, recognizing and neutralizing foreign substances.
- Motor proteins: Generate movement (e.g., myosin, kinesin).
- Receptor proteins: Bind to specific molecules and trigger cellular responses.
These are just a few examples of the vast array of functions that proteins perform, all stemming from their unique three-dimensional structures, which are ultimately determined by the sequence and interactions of their constituent amino acids. It's impossible to overstate the critical role of these intricate structures and the collaboration between amino acids within them.
Conclusion: The Collective Power of Amino Acids
In summary, no amino acid molecule by itself possesses significant biological activity. Their power lies in their ability to join together, forming peptide chains and intricate three-dimensional protein structures. The primary structure, dictated by the amino acid sequence, determines the secondary, tertiary, and quaternary structures, ultimately shaping the protein's function. The complexity of protein structure underlies the immense diversity of biological roles proteins play in living organisms. Understanding this fundamental concept is essential for appreciating the intricate mechanisms of life itself. The study of amino acids and proteins continues to be a vibrant and essential area of research, constantly revealing new insights into the complex mechanisms of life and offering new avenues for technological innovation in medicine, biotechnology, and materials science.
Latest Posts
Latest Posts
-
According To The Chart When Did A Pdsa Cycle Occur
May 12, 2025
-
Bioflix Activity Gas Exchange The Respiratory System
May 12, 2025
-
Economic Value Creation Is Calculated As
May 12, 2025
-
Which Items Typically Stand Out When You Re Scanning Text
May 12, 2025
-
Assume That Price Is An Integer Variable
May 12, 2025
Related Post
Thank you for visiting our website which covers about No Amino Acid Molecule By Itself . We hope the information provided has been useful to you. Feel free to contact us if you have any questions or need further assistance. See you next time and don't miss to bookmark.