Part 3 Comparing Model Vs Real Molecules
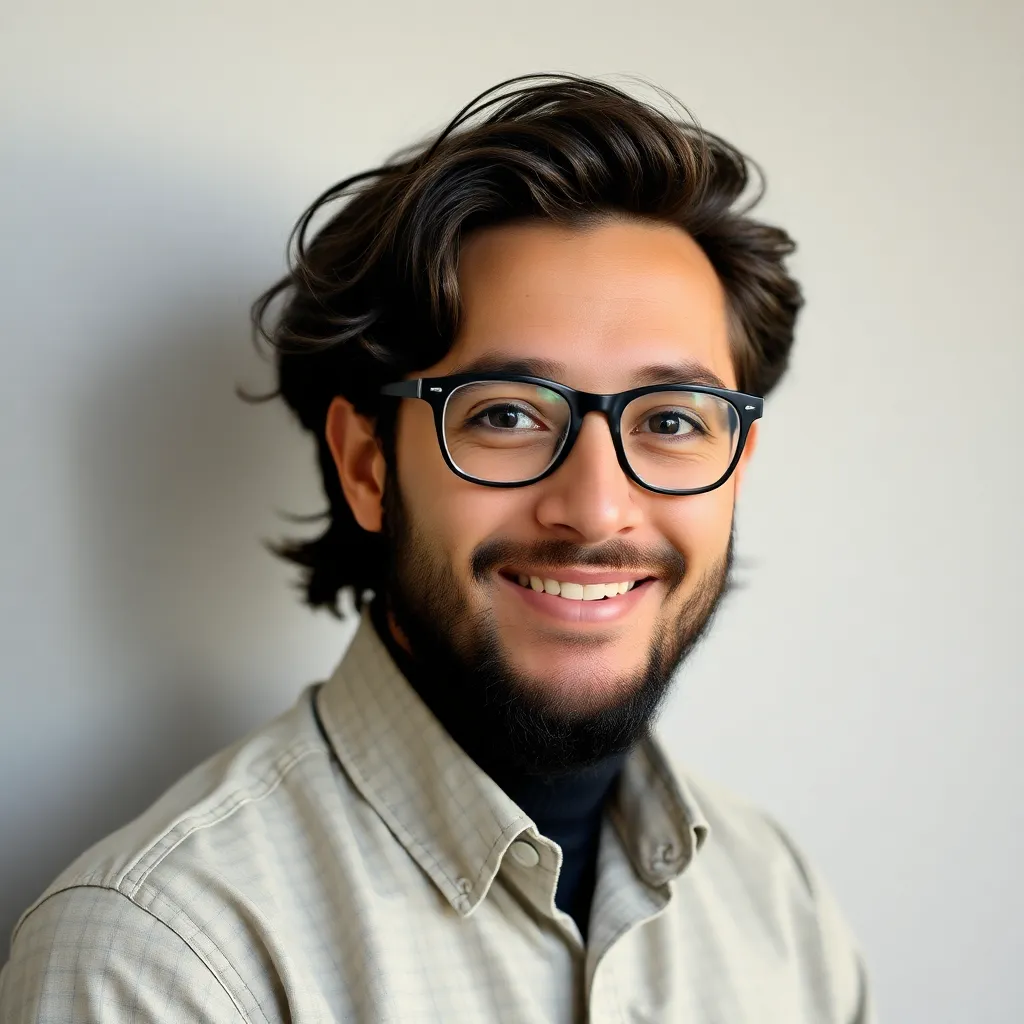
Onlines
May 10, 2025 · 6 min read
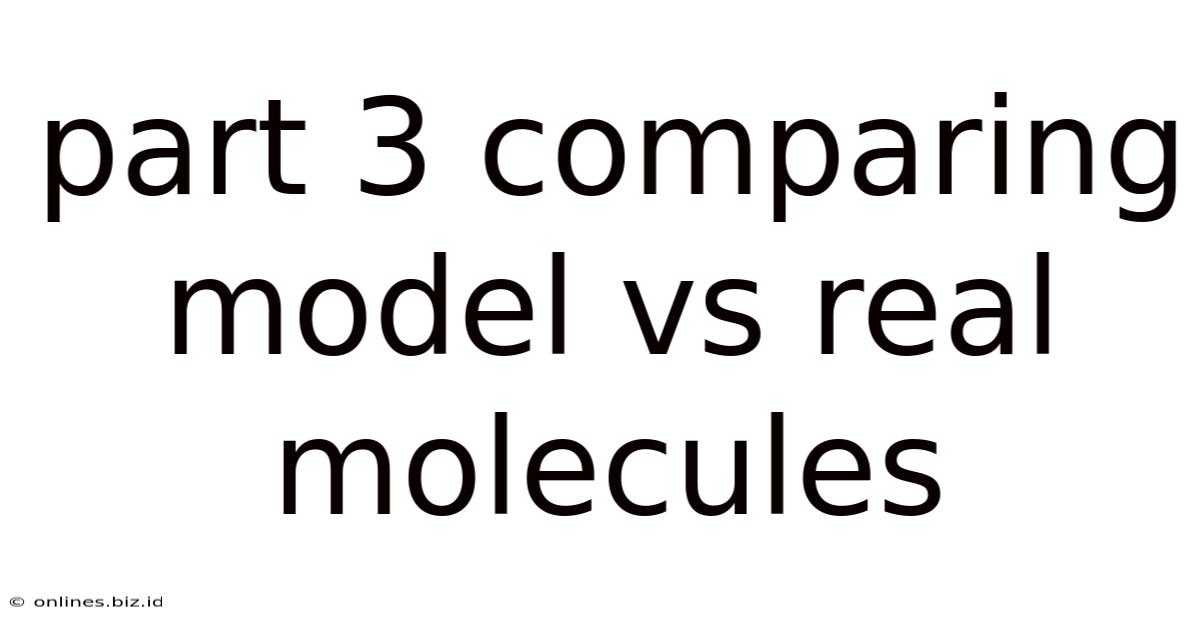
Table of Contents
Part 3: Comparing Model vs. Real Molecules: A Deep Dive into Molecular Representation
The journey of understanding molecules begins with representation. From simple ball-and-stick models to sophisticated computational simulations, the ways we depict molecules profoundly impact our comprehension of their properties and behavior. This third part delves deeper into the comparison between model and real molecules, focusing on the strengths and limitations of different modeling techniques and the implications for various scientific disciplines.
The Spectrum of Molecular Models: From Simple to Complex
Molecular models are not one-size-fits-all. Their complexity and accuracy vary widely, depending on the intended application and the level of detail required.
1. Ball-and-Stick Models: These are the most basic representations, using spheres to represent atoms and sticks to depict bonds. While incredibly simple and intuitive for visualizing basic molecular geometry, they fail to accurately portray crucial aspects like bond angles, electron densities, and intermolecular forces. Their primary advantage lies in their ease of construction and understanding, making them ideal for introductory teaching and basic conceptualization.
2. Space-Filling Models: These models offer a more realistic representation by showing the relative sizes of atoms. Spheres representing atoms are scaled to their van der Waals radii, giving a better visualization of molecular volume and how atoms might interact in three-dimensional space. However, they still lack the detail of electron distribution and don't explicitly show bond types.
3. Calotte Models: Improving upon space-filling models, calotte models use overlapping spherical segments to represent atoms, providing a smoother surface that more closely resembles the electron cloud. These models are particularly useful for visualizing surface areas and potential interaction sites.
4. Computational Models: These advanced representations use sophisticated algorithms and quantum mechanics to simulate molecular behavior. Software packages like Gaussian, GAMESS, and others enable researchers to calculate electronic structures, predict molecular properties like bond lengths and angles, and simulate dynamic processes like molecular vibrations and reactions. These models offer significantly greater accuracy compared to physical models but require substantial computational resources and expertise.
Bridging the Gap: From Model to Reality
While molecular models offer valuable insights, it's crucial to acknowledge their inherent limitations. No model perfectly replicates the intricate complexity of a real molecule. The discrepancies between model and reality stem from various factors:
1. Simplifications and Approximations: All models involve simplifications. Computational models, for instance, often employ approximations in their calculations to reduce computational costs. These approximations might compromise accuracy, especially for large and complex molecules.
2. Static vs. Dynamic Behavior: Many models represent molecules in a static state, neglecting their dynamic nature. Real molecules are constantly vibrating, rotating, and undergoing conformational changes. While some sophisticated computational models incorporate dynamic simulations, understanding this dynamic behavior remains a significant challenge.
3. Quantum Effects: The behavior of electrons in real molecules is governed by quantum mechanics. Accurately modeling these quantum effects is computationally demanding and often requires significant approximations. This can lead to discrepancies in predicting certain properties, particularly those highly sensitive to electron distribution.
4. Environmental Effects: Real molecules exist within a specific environment, which can influence their behavior. Solvent effects, intermolecular interactions, and temperature all play a role. While some models incorporate environmental factors, the accurate representation of these complexities remains a major challenge.
The Impact of Model Accuracy on Different Fields
The choice of molecular model significantly impacts the accuracy and reliability of results across various scientific disciplines.
1. Pharmaceutical Research and Drug Design: Accurate molecular models are crucial for understanding drug-receptor interactions. Computational modeling enables the prediction of drug efficacy and potential side effects, allowing researchers to design more effective and safer drugs. The accuracy of these predictions directly impacts the success rate of drug development.
2. Materials Science and Engineering: Molecular modeling plays a significant role in designing new materials with desired properties. From predicting the strength of a polymer to understanding the catalytic activity of a material, accurate models are essential for guiding materials development. Imperfect models can lead to inaccurate predictions, potentially wasting resources and delaying the development process.
3. Biochemistry and Enzymology: Understanding enzyme mechanisms and protein-ligand interactions requires accurate molecular models. These models enable researchers to study enzyme kinetics, predict reaction pathways, and design enzyme inhibitors. The accuracy of these models directly impacts our ability to develop new therapies for various diseases.
4. Environmental Science: Accurate molecular models are essential for studying environmental pollutants and predicting their behavior in the environment. Modeling the transport, fate, and toxicity of pollutants helps researchers develop strategies for pollution mitigation and remediation. Inaccurate models could lead to flawed environmental policies and ineffective remediation strategies.
Advanced Modeling Techniques and Future Directions
The field of molecular modeling is constantly evolving. Several advanced techniques are emerging that aim to improve the accuracy and reliability of molecular representations.
1. Density Functional Theory (DFT): DFT is a widely used computational method that provides a good balance between accuracy and computational cost. It's particularly valuable for studying the electronic structure and properties of molecules.
2. Molecular Dynamics (MD): MD simulations allow researchers to study the dynamic behavior of molecules over time. These simulations provide insights into molecular motions, conformational changes, and interactions.
3. Quantum Monte Carlo (QMC): QMC methods provide highly accurate results but are computationally expensive. They are particularly useful for studying systems where correlation effects are significant.
4. Machine Learning (ML): ML techniques are increasingly used in molecular modeling to improve the accuracy of predictions and accelerate the simulation process. ML models can learn from existing data to predict molecular properties and behaviors.
5. Multiscale Modeling: This approach combines different modeling techniques to study systems at multiple scales. For instance, it might combine quantum mechanical calculations for a specific part of a system with classical MD simulations for the rest of the system. This hybrid approach allows researchers to study complex systems more efficiently while maintaining accuracy.
Conclusion: A Continuous Pursuit of Accuracy
The gap between model and reality in molecular representation is a continuous challenge. While current models offer valuable insights and predictions, the pursuit of greater accuracy remains a central theme in scientific research. Ongoing advances in computational methods, algorithms, and hardware are gradually bridging this gap. The development of more sophisticated models, incorporating advanced techniques like ML and multiscale modeling, will continue to revolutionize our understanding of molecules and their behavior, ultimately leading to transformative advancements across multiple scientific disciplines. The future lies in ever-more accurate and comprehensive representations that faithfully capture the intricate dance of atoms and electrons in the real world. The continuous refinement of molecular models will not only enhance our fundamental understanding of molecular systems but also enable us to design and engineer novel materials and therapies with unprecedented precision and efficiency.
Latest Posts
Latest Posts
-
Because Adult Cognition Combines Logic And Experience It Is Considered
May 10, 2025
-
A Desperate Hiker Has To Think Fast
May 10, 2025
-
Carl Rogerss Position On Confronting The Client Is That
May 10, 2025
-
Www Mathworksheets4kids Com Find The Slope Answer Key
May 10, 2025
-
Yellow Wind Sage Journey To The West
May 10, 2025
Related Post
Thank you for visiting our website which covers about Part 3 Comparing Model Vs Real Molecules . We hope the information provided has been useful to you. Feel free to contact us if you have any questions or need further assistance. See you next time and don't miss to bookmark.