Photosynthesis What's In A Leaf Answer Key
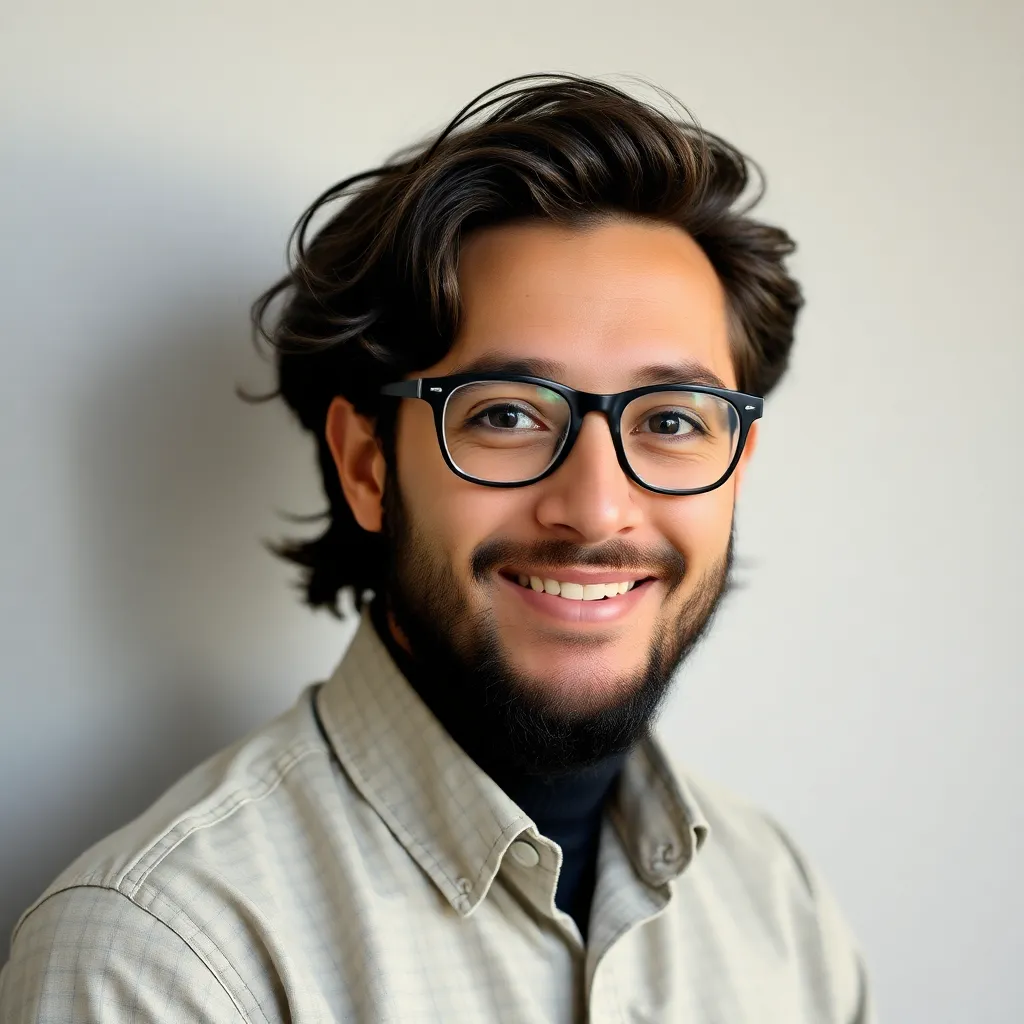
Onlines
May 08, 2025 · 7 min read
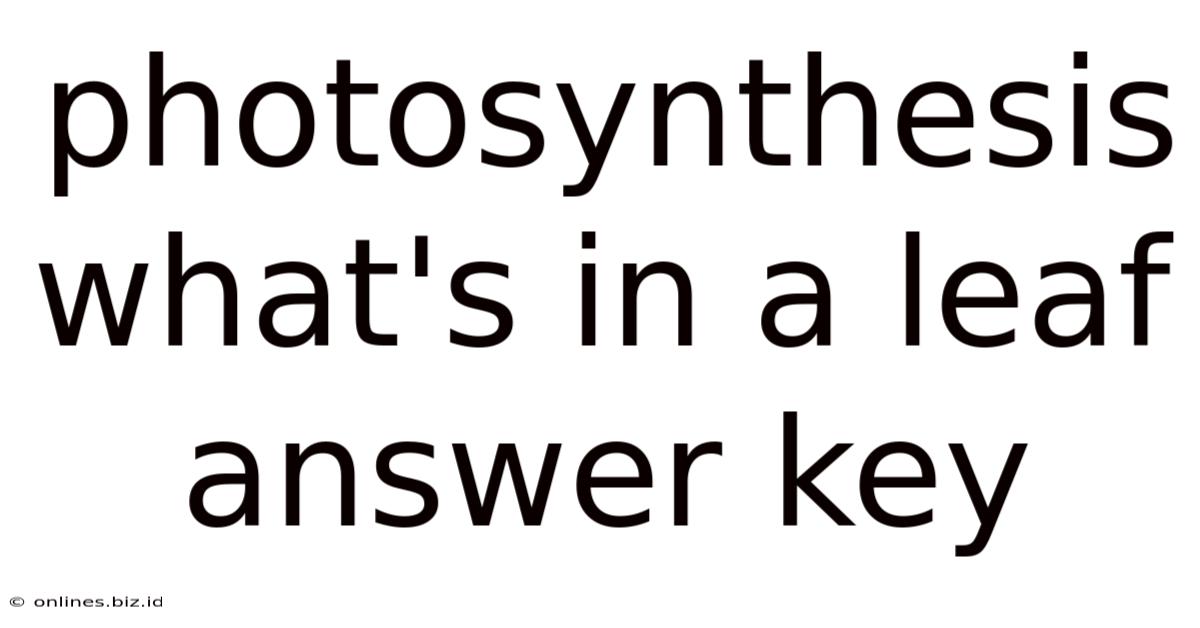
Table of Contents
Photosynthesis: What's in a Leaf? Answer Key
Photosynthesis, the remarkable process by which plants convert sunlight into energy, is fundamental to life on Earth. Understanding this process requires delving into the intricate structure of a leaf, the plant's primary photosynthetic organ. This article serves as a comprehensive "answer key," exploring the key components within a leaf and their roles in the magic of photosynthesis.
The Leaf: A Photosynthetic Powerhouse
Leaves are nature's solar panels, meticulously designed to capture sunlight and facilitate the biochemical reactions of photosynthesis. Their structure is far from simple; it's a marvel of evolutionary engineering optimized for light absorption, gas exchange, and water conservation. Let's dissect the key players within:
1. The Epidermis: Protection and Regulation
The leaf's outer layer, the epidermis, acts as a protective shield. It's composed of tightly packed epidermal cells, which prevent water loss and protect the inner tissues from pathogens and physical damage. However, the epidermis isn't completely impermeable. Specialized cells called guard cells flank tiny pores known as stomata. These stomata are crucial for gas exchange—allowing carbon dioxide (CO2) to enter the leaf and oxygen (O2) and water vapor to exit. The regulation of stomata opening and closing is a finely tuned process, influenced by factors like light intensity, temperature, and humidity, ensuring efficient photosynthesis while minimizing water loss through transpiration. This delicate balance is crucial for plant survival, especially in arid environments. A waxy cuticle covering the epidermis further reduces water loss. The cuticle, a hydrophobic layer, significantly limits transpiration, making it a crucial adaptation in dry climates. Differences in cuticle thickness and composition reflect the plant's adaptation to its specific environment.
2. The Mesophyll: The Photosynthetic Factory
Beneath the epidermis lies the mesophyll, the leaf's photosynthetic powerhouse. This tissue is composed of two main types of cells:
-
Palisade mesophyll: These elongated cells are densely packed, particularly in the upper part of the leaf, maximizing light absorption. They contain numerous chloroplasts, the organelles responsible for photosynthesis. Their columnar arrangement ensures efficient light interception, with sunlight penetrating deep into the leaf tissue. The arrangement of palisade cells is crucial for maximizing light capture, particularly in sun-loving plants. Plants adapted to shade often have fewer layers of palisade cells.
-
Spongy mesophyll: Located below the palisade mesophyll, the spongy mesophyll cells are loosely arranged, creating air spaces between them. These spaces facilitate the diffusion of gases (CO2 and O2) within the leaf, ensuring that carbon dioxide reaches the chloroplasts efficiently. The spongy mesophyll’s structure facilitates gas exchange, with the large intercellular spaces connecting to the stomata, creating an internal network for gas movement. The efficiency of this system is critical for the continuous supply of CO2 necessary for photosynthesis.
3. Chloroplasts: The Photosynthetic Organelles
Within both palisade and spongy mesophyll cells, we find chloroplasts, the tiny organelles responsible for the actual photosynthetic process. These are not simply containers; they are highly organized structures with multiple components crucial for capturing and converting light energy:
-
Thylakoid membranes: These are flattened sacs within the chloroplast, arranged in stacks called grana. Embedded within the thylakoid membranes are chlorophyll molecules and other pigments that capture light energy. The thylakoid membrane’s structure, with its intricate arrangement of proteins and pigments, is critical for the light-dependent reactions of photosynthesis.
-
Stroma: The fluid-filled space surrounding the thylakoids is called the stroma. This is where the carbon fixation reactions of photosynthesis (the Calvin cycle) occur, converting CO2 into glucose. The stroma contains enzymes, DNA, and ribosomes, making it a highly active metabolic center. The stroma's role in the Calvin cycle is vital for producing the sugars that fuel plant growth and development.
-
Chlorophyll: This green pigment is the key player in light absorption. Different types of chlorophyll (a and b) absorb different wavelengths of light, maximizing the leaf's ability to capture the sun's energy. The specific ratios of chlorophyll a and b vary depending on the plant species and its light environment. Carotenoids and other accessory pigments absorb light at wavelengths not efficiently absorbed by chlorophyll, widening the spectrum of usable light for photosynthesis.
The Process of Photosynthesis: A Deeper Dive
Photosynthesis is a two-stage process:
1. The Light-Dependent Reactions: Capturing Light Energy
This stage takes place within the thylakoid membranes. Light energy is absorbed by chlorophyll and other pigments, exciting electrons. These energized electrons move along an electron transport chain, releasing energy that is used to generate ATP (adenosine triphosphate), the cell's energy currency, and NADPH, a reducing agent. Water is split (photolysis) in this process, releasing oxygen as a byproduct. This oxygen is what we breathe! The efficiency of the light-dependent reactions is crucial, determining how much energy is available for the subsequent carbon fixation steps. Environmental factors such as light intensity, temperature, and water availability significantly influence this stage.
2. The Light-Independent Reactions (Calvin Cycle): Building Sugars
This stage takes place in the stroma. The ATP and NADPH generated in the light-dependent reactions provide the energy and reducing power needed to convert carbon dioxide into glucose. This process, a complex series of enzymatic reactions, involves the fixation of CO2 by RuBisCO (ribulose-1,5-bisphosphate carboxylase/oxygenase), the most abundant enzyme on Earth. The resulting glucose is then used by the plant for energy, growth, and the synthesis of other organic molecules. The efficiency of the Calvin cycle is tightly regulated, influenced by factors like CO2 concentration, temperature, and the availability of ATP and NADPH. Variations in the Calvin cycle exist in different plant species, reflecting adaptations to different environments.
Factors Affecting Photosynthesis
Several factors influence the rate of photosynthesis:
-
Light intensity: Increased light intensity generally leads to increased photosynthesis, up to a saturation point. Beyond this point, further increases in light intensity have no effect, and may even damage the photosynthetic apparatus.
-
Carbon dioxide concentration: CO2 is a crucial reactant in photosynthesis. Increasing CO2 concentration can increase the rate of photosynthesis, but only up to a certain point, after which other factors become limiting.
-
Temperature: Enzymes involved in photosynthesis have optimal temperature ranges. Temperatures too high or too low can reduce enzyme activity and decrease the rate of photosynthesis.
-
Water availability: Water is essential for photosynthesis; its deficiency can reduce the rate of photosynthesis by limiting the supply of electrons for the light-dependent reactions and impacting stomatal function.
Variations in Photosynthesis: C3, C4, and CAM Plants
Different plants have evolved different photosynthetic strategies to adapt to their environments:
-
C3 plants: These are the most common type of plants, performing the Calvin cycle directly in mesophyll cells. They are generally more efficient at photosynthesis in cooler, wetter conditions. Examples include rice, wheat, and soybeans.
-
C4 plants: These plants have evolved a mechanism to concentrate CO2 around RuBisCO, reducing photorespiration (a wasteful process where RuBisCO uses oxygen instead of CO2). They are well-suited to hot, dry conditions. Examples include corn and sugarcane.
-
CAM plants: These plants, often found in arid environments, open their stomata at night to take in CO2 and store it as an acid. During the day, when the stomata are closed to conserve water, CO2 is released from the acid and used in photosynthesis. Examples include cacti and succulents.
Conclusion: The Intricate Beauty of Photosynthesis
The leaf, far from being a simple structure, is a complex and highly efficient photosynthetic organ. Understanding its internal components—the epidermis, mesophyll, and chloroplasts—and their roles in the light-dependent and light-independent reactions of photosynthesis reveals the remarkable ingenuity of nature. Factors influencing photosynthesis and the variations in photosynthetic strategies across plant species further highlight the adaptability and resilience of plant life. This "answer key" has provided a comprehensive overview of this fundamental process, emphasizing the key components and processes involved in transforming sunlight into energy, the very foundation of most terrestrial ecosystems. The intricacies of photosynthesis continue to be a subject of ongoing research, revealing new aspects of its complexity and importance to the planet's health and sustainability.
Latest Posts
Latest Posts
-
What Are Two Major Concerns Regarding Iot Devices Select Two
May 09, 2025
-
Which Planet Listed Below Has The Most Extreme Seasons
May 09, 2025
-
Co Locating Staff For A Project Is A Good Strategy To
May 09, 2025
-
Ap Environmental Science 2020 Practice Exam 1 Mcq
May 09, 2025
-
Gen Bio 115 Rutgers Exam 1
May 09, 2025
Related Post
Thank you for visiting our website which covers about Photosynthesis What's In A Leaf Answer Key . We hope the information provided has been useful to you. Feel free to contact us if you have any questions or need further assistance. See you next time and don't miss to bookmark.