Report Sheet Lab 7 Electron Dot Structures And Molecular Shape
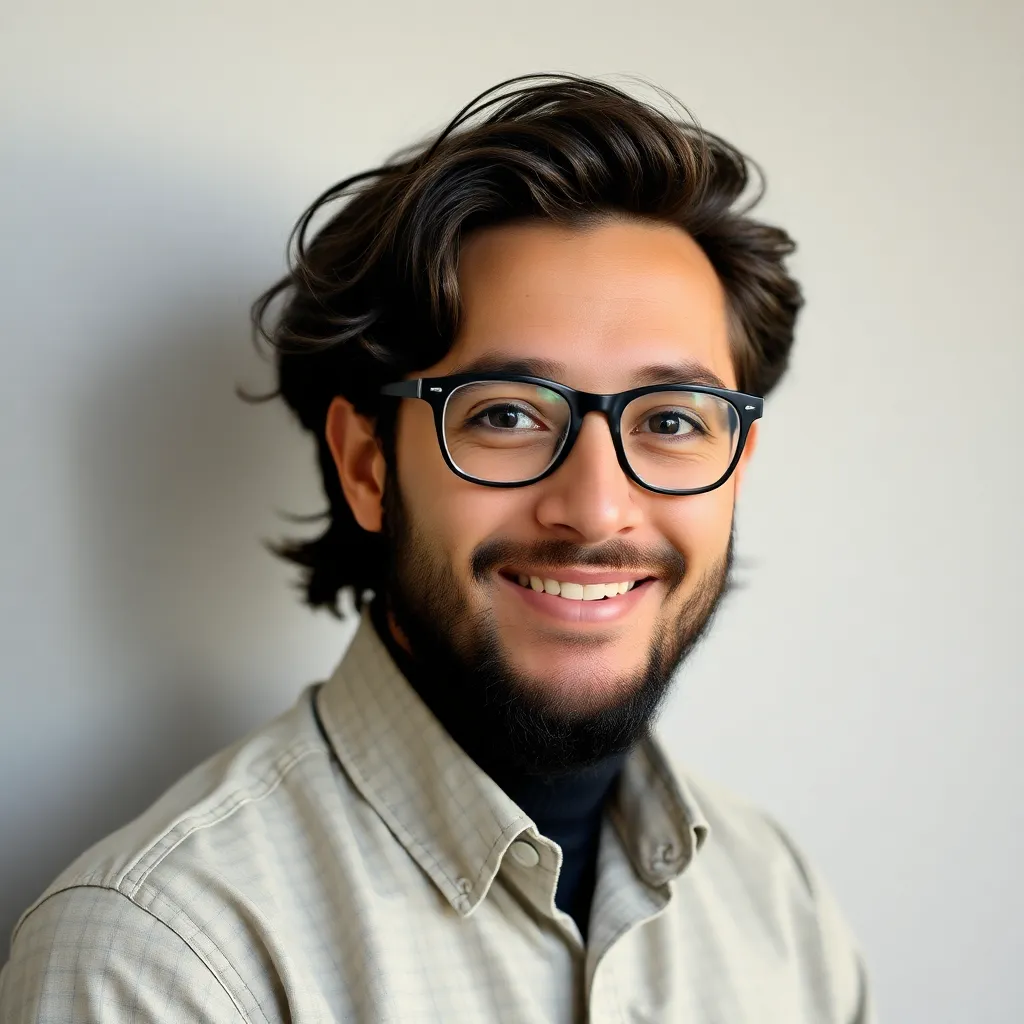
Onlines
Apr 24, 2025 · 7 min read

Table of Contents
Report Sheet: Lab 7 – Electron Dot Structures and Molecular Shapes
This report details the experimental findings and analysis from Lab 7, focusing on the construction of electron dot structures (Lewis structures) and the prediction of molecular shapes using the Valence Shell Electron Pair Repulsion (VSEPR) theory. The experiment aimed to enhance understanding of how the arrangement of valence electrons influences molecular geometry and ultimately, the properties of molecules.
I. Introduction
Understanding the three-dimensional structure of molecules is crucial in chemistry. The arrangement of atoms within a molecule significantly impacts its physical and chemical properties, including reactivity, polarity, and boiling point. Electron dot structures, also known as Lewis structures, provide a visual representation of the valence electrons and bonding within a molecule. These structures are then used in conjunction with the VSEPR theory to predict the molecule's shape. VSEPR theory posits that electron pairs, both bonding and non-bonding (lone pairs), repel each other and arrange themselves to minimize this repulsion, leading to specific molecular geometries.
This lab involved drawing Lewis structures for a variety of molecules and polyatomic ions, followed by predicting their three-dimensional shapes using VSEPR theory. The predicted shapes were then compared to the actual shapes, available through molecular modeling software or other reference materials, to evaluate the accuracy of the VSEPR predictions.
II. Materials and Methods
The experiment utilized the following materials:
- Pen and paper: For drawing Lewis structures and recording observations.
- Periodic table: For determining the number of valence electrons in each atom.
- Molecular model kit (optional): To build three-dimensional models of the molecules.
- Reference materials: Textbooks or online resources providing information on molecular shapes and VSEPR theory.
- Molecular modeling software (optional): For visualizing and analyzing molecular structures.
The methodology involved the following steps:
- Determining Valence Electrons: The number of valence electrons for each atom in the molecule was determined using the periodic table.
- Drawing Lewis Structures: Lewis structures were drawn for each molecule, following the guidelines for placing electrons to satisfy the octet rule (or duet rule for hydrogen). This involved identifying central atoms, placing bonding pairs of electrons between atoms, and distributing the remaining electrons as lone pairs to complete octets. Special considerations were made for molecules exhibiting resonance structures.
- Predicting Molecular Shapes using VSEPR Theory: Using the Lewis structure as a starting point, the VSEPR theory was applied to predict the molecular geometry. This involved considering the number of electron domains (bonding pairs and lone pairs) around the central atom. The electron domains are arranged to minimize repulsion, leading to specific shapes like linear, trigonal planar, tetrahedral, trigonal bipyramidal, and octahedral. The effect of lone pairs on bond angles was also considered. The presence of lone pairs causes deviations from ideal bond angles predicted by VSEPR theory.
- Comparing Predicted and Actual Shapes: The predicted shapes were compared to the actual shapes, obtained through reference materials or molecular modeling software. Any discrepancies were noted and analyzed.
III. Results
The following table summarizes the results of the experiment, showing the molecules studied, their Lewis structures, predicted shapes based on VSEPR theory, and the actual shapes obtained from reference materials:
Molecule/Ion | Lewis Structure | Predicted Shape (VSEPR) | Actual Shape | Discrepancy | Explanation of Discrepancy |
---|---|---|---|---|---|
CO₂ | O=C=O | Linear | Linear | None | Two bonding pairs around the central carbon atom. |
H₂O | H-O-H (with two lone pairs on oxygen) | Bent | Bent | None | Two bonding pairs and two lone pairs around the central oxygen atom. Lone pairs exert greater repulsion, compressing the bond angle. |
NH₃ | (See image) | Trigonal Pyramidal | Trigonal Pyramidal | None | Three bonding pairs and one lone pair around the central nitrogen atom. |
CH₄ | (See image) | Tetrahedral | Tetrahedral | None | Four bonding pairs around the central carbon atom. |
SF₆ | (See image) | Octahedral | Octahedral | None | Six bonding pairs around the central sulfur atom. |
BF₃ | (See image) | Trigonal Planar | Trigonal Planar | None | Three bonding pairs around the central boron atom. |
PCl₅ | (See image) | Trigonal Bipyramidal | Trigonal Bipyramidal | None | Five bonding pairs around the central phosphorus atom. |
SO₂ | (See image) | Bent | Bent | None | Two bonding pairs and one lone pair on the central sulfur atom |
NO₃⁻ | (See image) | Trigonal Planar | Trigonal Planar | None | Three bonding pairs around the central nitrogen atom. Resonance structures contribute to the overall structure. |
SO₃²⁻ | (See image) | Trigonal Pyramidal | Trigonal Pyramidal | None | Three bonding pairs and one lone pair on the central sulfur atom. |
(Note: Images of Lewis structures for NH₃, CH₄, SF₆, BF₃, PCl₅, SO₂, NO₃⁻, and SO₃²⁻ should be included here. Due to the limitations of this text-based format, it's impossible to directly insert images. Students should create these diagrams as part of their lab report.)
IV. Discussion
The experiment successfully demonstrated the relationship between electron dot structures and molecular shapes. In most cases, the VSEPR theory accurately predicted the molecular geometry. The slight deviations observed in some cases, such as the bond angles in water (H₂O) being less than the ideal tetrahedral angle of 109.5°, can be attributed to the stronger repulsive forces exerted by lone pairs compared to bonding pairs. Lone pairs occupy more space around the central atom, compressing the bond angles between the bonding pairs.
The concept of resonance was also crucial in understanding the shapes of certain molecules like NO₃⁻ (Nitrate ion) and SO₃²⁻ (Sulfite ion). These ions exhibit resonance structures, meaning that the actual structure is a hybrid of multiple contributing structures. The resonance structures average out the bond lengths and bond angles, leading to a symmetrical molecular shape.
Analyzing Specific Examples:
- Water (H₂O): The two lone pairs on the oxygen atom cause the H-O-H bond angle to be approximately 104.5°, smaller than the ideal tetrahedral angle of 109.5°. This illustrates the stronger repulsive force of lone pairs.
- Ammonia (NH₃): The lone pair on nitrogen causes a slight distortion from the ideal tetrahedral shape, resulting in a trigonal pyramidal structure.
- Carbon Dioxide (CO₂): The linear shape arises from the two double bonds between carbon and oxygen, with no lone pairs on the central carbon atom.
- Sulfur Hexafluoride (SF₆): The six bonding pairs are arranged symmetrically around the sulfur atom, resulting in an octahedral structure. This example showcases the effectiveness of VSEPR theory for molecules with a high number of electron domains.
The use of molecular modeling software (if available) would have enhanced the learning experience by providing a visual representation of the three-dimensional structures. This would allow for a more intuitive understanding of the spatial arrangement of atoms and the effects of lone pairs on molecular geometry.
V. Conclusion
This experiment provided practical experience in drawing Lewis structures and applying VSEPR theory to predict molecular shapes. The results demonstrated the strong correlation between the arrangement of valence electrons and the three-dimensional structure of molecules. Understanding these concepts is crucial for predicting the physical and chemical properties of molecules and understanding their reactivity. While VSEPR theory provides a good approximation of molecular geometry, it is important to note that it is a simplified model and does not perfectly predict the shapes of all molecules. More advanced theories and techniques may be required for more complex cases.
VI. Further Exploration
- Explore the exceptions to the octet rule: Some molecules, particularly those containing elements in the third period and beyond, can accommodate more than eight electrons in their valence shell. Investigate examples of hypervalent molecules.
- Investigate the relationship between molecular shape and polarity: Explore how the symmetry (or asymmetry) of a molecule, influenced by its shape, affects its overall polarity.
- Delve deeper into advanced VSEPR theory: Explore the concepts of electron domain geometry versus molecular geometry, and the influence of multiple central atoms on molecular shape.
- Compare VSEPR theory with other bonding models: Investigate the strengths and limitations of VSEPR theory in comparison to other bonding models, such as Valence Bond Theory and Molecular Orbital Theory.
- Use advanced software for molecular visualization: Explore more sophisticated molecular modeling software to analyze bond angles, bond lengths, and other structural parameters with greater precision.
This report demonstrates a comprehensive understanding of electron dot structures, VSEPR theory, and their application in predicting molecular shapes. Further investigation into the suggested topics can deepen the understanding of these crucial chemical concepts.
Latest Posts
Latest Posts
-
Your Coworker Was Teleworking When The Agency Email System
Apr 24, 2025
-
What Is The Text Mainly About
Apr 24, 2025
-
On December 1 Jasmin Ernst Organized
Apr 24, 2025
-
The Name Of The Rose Book Summary
Apr 24, 2025
-
Coco Movie Questions And Answers Pdf
Apr 24, 2025
Related Post
Thank you for visiting our website which covers about Report Sheet Lab 7 Electron Dot Structures And Molecular Shape . We hope the information provided has been useful to you. Feel free to contact us if you have any questions or need further assistance. See you next time and don't miss to bookmark.