This Image Shows The Tertiary Structure Of A Protein Segment
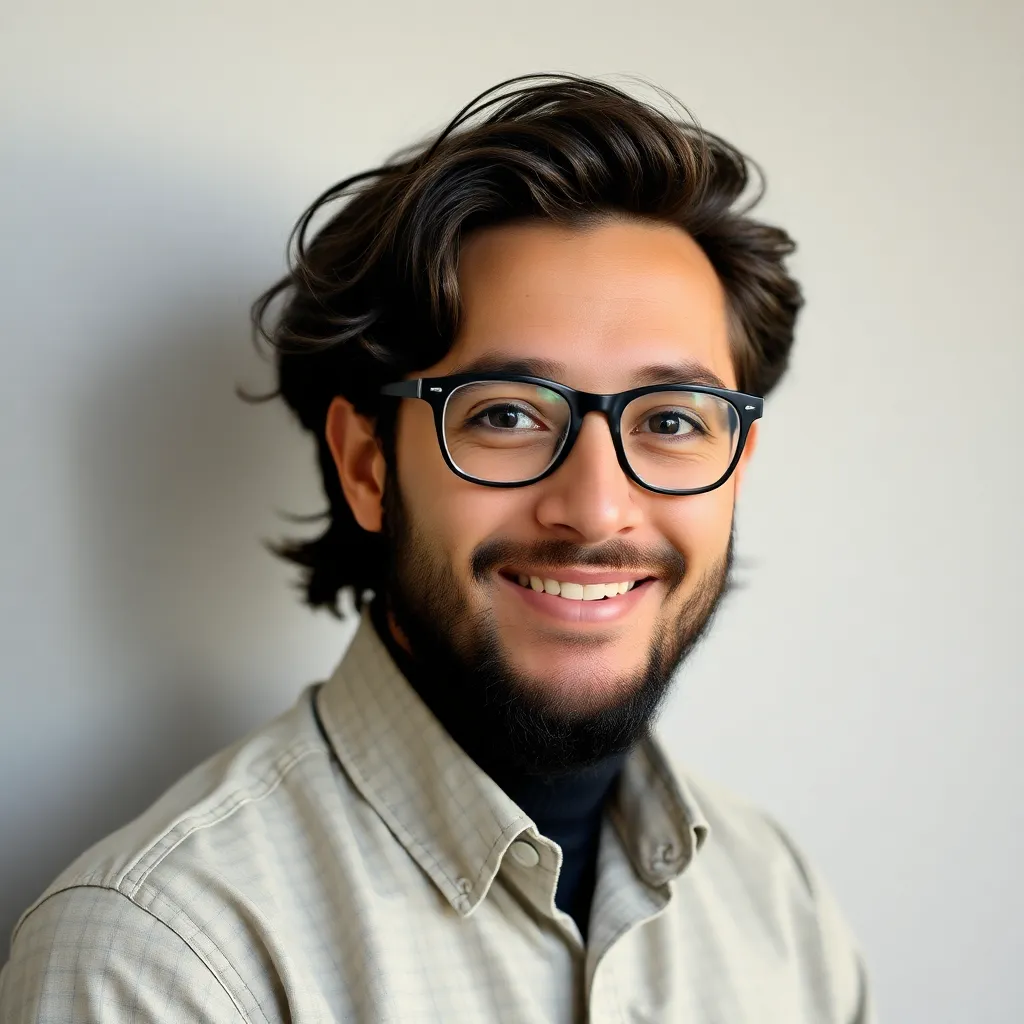
Onlines
Mar 31, 2025 · 6 min read

Table of Contents
Decoding the Tertiary Structure: A Deep Dive into Protein Folding
This image depicts a protein segment's tertiary structure, a crucial aspect of its function. Understanding this intricate three-dimensional arrangement is key to comprehending how proteins perform their diverse roles within living organisms. This article will explore the intricacies of tertiary protein structure, delving into the forces that shape it, its significance in biological processes, and the implications of its misfolding.
What is Tertiary Structure?
The tertiary structure of a protein refers to its three-dimensional conformation, arising from the folding of its polypeptide chain. This folding isn't random; it's meticulously orchestrated by various interactions between amino acid side chains, resulting in a unique and functional structure. Unlike secondary structures (alpha-helices and beta-sheets), which describe local folding patterns, tertiary structure encompasses the overall arrangement of the entire polypeptide chain. Think of secondary structures as the building blocks, while the tertiary structure is the magnificent architectural masterpiece they form.
Forces Shaping the Tertiary Structure:
Several non-covalent interactions and covalent bonds play crucial roles in stabilizing the tertiary structure:
-
Hydrophobic Interactions: Amino acid residues with nonpolar side chains tend to cluster together in the protein's core, away from the surrounding aqueous environment. This hydrophobic effect is a major driving force in protein folding.
-
Hydrogen Bonds: Hydrogen bonds form between polar side chains and/or the peptide backbone, contributing to the stability of the folded structure. These bonds are numerous and crucial in maintaining specific conformations.
-
Ionic Bonds (Salt Bridges): Electrostatic attractions between oppositely charged amino acid side chains (e.g., negatively charged aspartate and positively charged lysine) stabilize the tertiary structure. These interactions are particularly strong in the protein's interior.
-
Disulfide Bonds: Covalent bonds formed between cysteine residues (containing sulfhydryl groups) are strong and essential for stabilizing the tertiary structure, particularly in secreted proteins. These bonds are often strategically positioned to create loops or link distant parts of the polypeptide chain.
-
Van der Waals Forces: Weak interactions between atoms in close proximity contribute cumulatively to the overall stability of the folded protein. While individually weak, their collective effect is significant.
These forces work in concert to create a specific, tightly packed arrangement that minimizes free energy and maximizes stability. The precise balance and interplay of these forces determine the final three-dimensional shape of the protein.
The Significance of Tertiary Structure in Biological Function:
The tertiary structure is inextricably linked to a protein's function. The precise arrangement of amino acid residues determines the protein's:
-
Active Site: In enzymes, the tertiary structure precisely positions catalytic amino acid residues to form the active site, where substrate binding and catalysis occur. Even minor alterations in the tertiary structure can drastically affect catalytic efficiency.
-
Binding Sites: Proteins often interact with other molecules (ligands, other proteins, DNA, etc.). The tertiary structure creates specific binding sites with unique geometries and chemical properties that dictate the specificity and strength of these interactions.
-
Structural Roles: Many proteins contribute to the structural integrity of cells and tissues. Their tertiary structure provides the mechanical strength and stability needed to perform these structural roles, maintaining cell shape and integrity. Collagen, for example, has a unique triple-helix tertiary structure crucial for its structural function in connective tissues.
-
Transport and Storage: Proteins involved in transporting molecules (e.g., hemoglobin) or storing molecules (e.g., ferritin) rely on their tertiary structure to create pockets or channels for binding and transporting their cargo. The precise architecture of these binding sites ensures specificity and efficient transport or storage.
-
Signaling and Regulation: Many proteins act as signaling molecules or regulators of cellular processes. Their tertiary structure is crucial in determining their interaction with receptors or other signaling molecules, allowing for precise and regulated cellular communication.
Protein Folding and the Role of Chaperones:
The process of protein folding from a linear polypeptide chain to its functional tertiary structure is a complex and fascinating process. While the amino acid sequence dictates the final three-dimensional arrangement, the path towards this structure is not always straightforward. Many proteins require assistance from molecular chaperones, proteins that facilitate proper folding and prevent aggregation.
Chaperones bind to unfolded or partially folded proteins, preventing them from aggregating and potentially misfolding. They provide a protective environment that allows the protein to fold correctly, ensuring the formation of the functional tertiary structure. The failure of chaperones to properly assist in protein folding can have serious consequences.
Consequences of Misfolding:
Incorrect folding of proteins can lead to various adverse effects, including:
-
Loss of Function: A misfolded protein may lack the proper active site or binding site, rendering it non-functional. This can have severe consequences, depending on the protein's role in the cell or organism.
-
Aggregation: Misfolded proteins can aggregate, forming insoluble clumps that can damage cells and tissues. These aggregates are implicated in various diseases, including Alzheimer's disease, Parkinson's disease, and Huntington's disease. The accumulation of these protein aggregates disrupts normal cellular processes, leading to cellular dysfunction and disease pathology.
-
Dominant-Negative Effects: In some cases, a misfolded protein can interfere with the function of its correctly folded counterparts. This "dominant-negative" effect can amplify the severity of the consequences of protein misfolding.
-
Disease: Many diseases are directly linked to protein misfolding, including cystic fibrosis (due to misfolding of the CFTR protein), Alzheimer's disease (due to aggregation of amyloid-beta plaques), and Parkinson's disease (due to aggregation of alpha-synuclein). The study of protein misfolding is therefore crucial for developing therapeutic strategies for these debilitating diseases.
Techniques for Studying Tertiary Structure:
Various experimental techniques are used to determine the tertiary structure of proteins:
-
X-ray Crystallography: This method involves crystallizing the protein and then bombarding the crystals with X-rays. The diffraction pattern produced allows researchers to deduce the three-dimensional structure of the protein. This technique provides high-resolution structural information but requires the protein to be crystallized, which can be challenging.
-
Nuclear Magnetic Resonance (NMR) Spectroscopy: This technique uses magnetic fields to study the interactions between atomic nuclei in the protein. The data obtained can be used to determine the protein's three-dimensional structure in solution. NMR spectroscopy is particularly useful for studying proteins that are difficult to crystallize.
-
Cryo-Electron Microscopy (Cryo-EM): Cryo-EM is a powerful technique that allows for the determination of protein structures at near-atomic resolution, without the need for crystallization. It's rapidly becoming a dominant technique in structural biology, allowing the study of large macromolecular complexes and intrinsically disordered proteins.
Conclusion:
The tertiary structure of a protein is a crucial determinant of its biological function. The intricate interplay of various forces shapes this three-dimensional architecture, resulting in precisely positioned active sites, binding sites, and structural features essential for the protein's role in cellular processes. Understanding protein folding, the factors that influence it, and the consequences of misfolding is paramount not only for basic biological research but also for developing therapeutic strategies to combat diseases associated with protein misfolding. Continued advancements in structural biology techniques will further refine our understanding of protein structure-function relationships and pave the way for novel therapeutic interventions. The image provided, a snapshot of this complex folding, serves as a powerful reminder of the intricacies and significance of the tertiary structure in the grand scheme of life.
Latest Posts
Latest Posts
-
A Technician Is Explaining The Difference Between
Apr 02, 2025
-
Summary Of Chapter 2 The Scarlet Letter
Apr 02, 2025
-
An Animal Will Gain Weight When
Apr 02, 2025
-
Characters Of The Lottery Shirley Jackson
Apr 02, 2025
-
All Of The Following Are Fraudulent Health Care Practices Except
Apr 02, 2025
Related Post
Thank you for visiting our website which covers about This Image Shows The Tertiary Structure Of A Protein Segment . We hope the information provided has been useful to you. Feel free to contact us if you have any questions or need further assistance. See you next time and don't miss to bookmark.