Which Of The Following Materials May Form Crystalline Solids
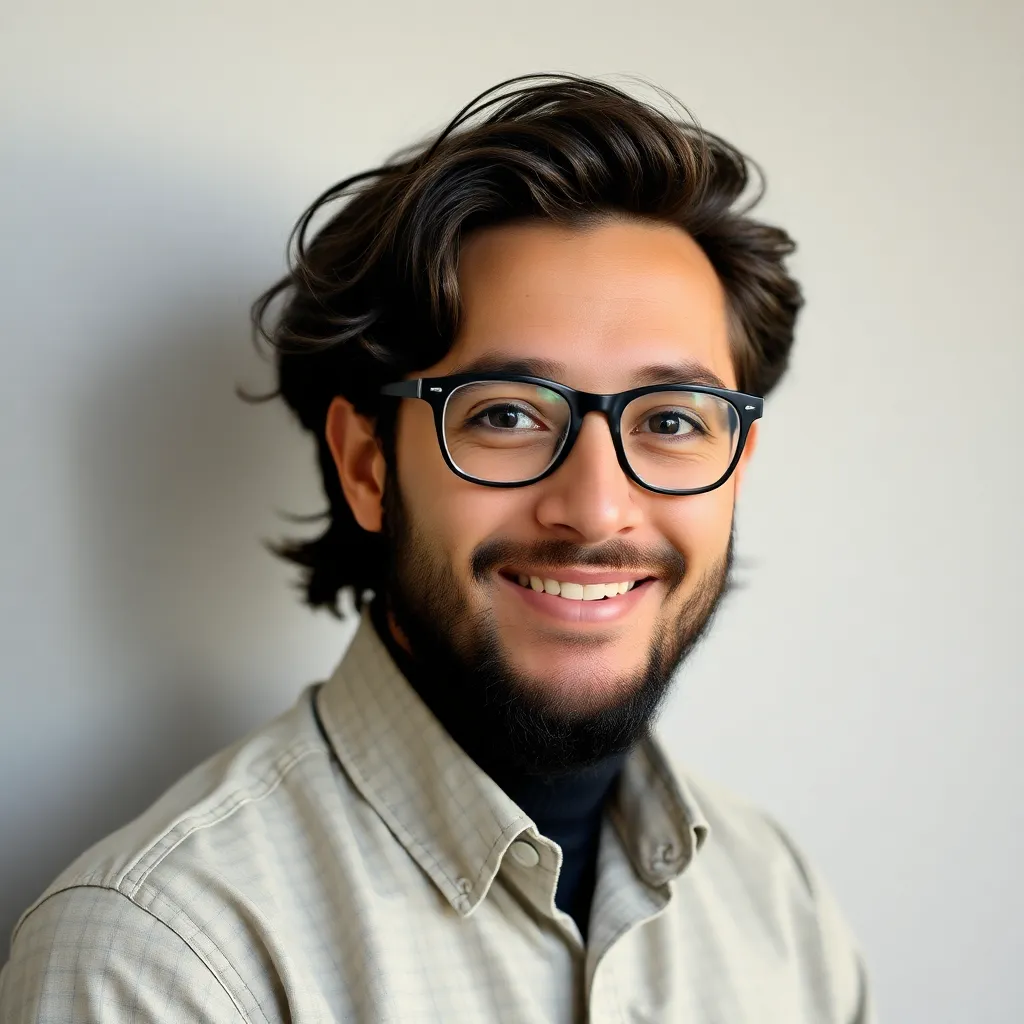
Onlines
May 07, 2025 · 7 min read
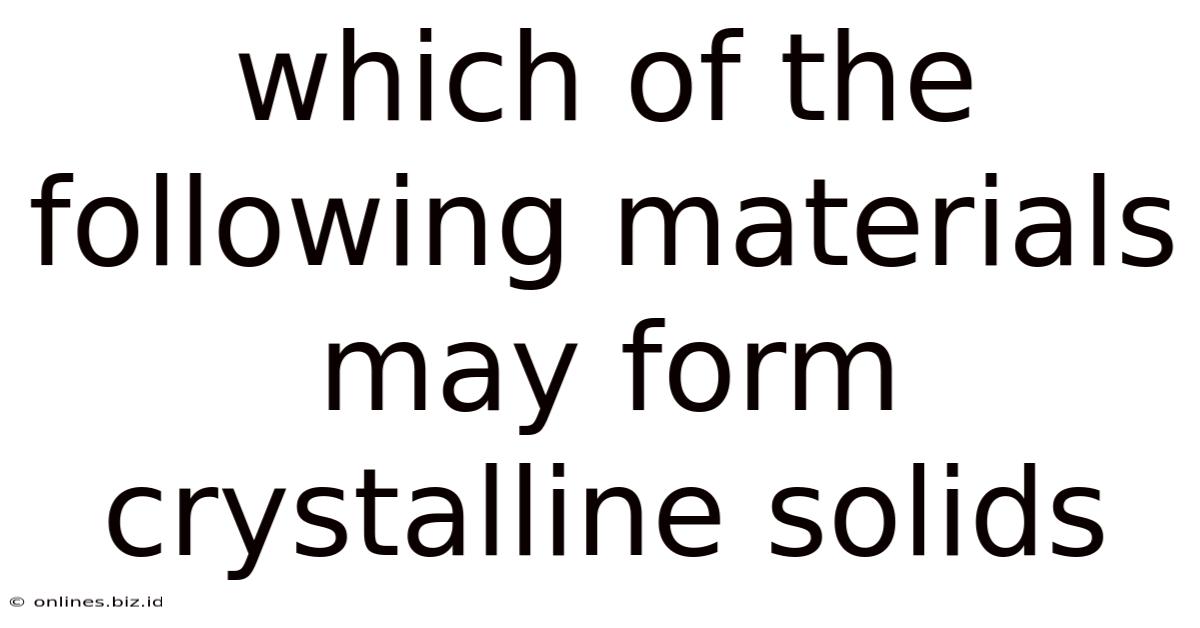
Table of Contents
Which of the Following Materials May Form Crystalline Solids?
Crystalline solids are a fascinating class of materials characterized by their highly ordered, repeating three-dimensional atomic arrangement. Understanding which materials can form these structures is crucial in various fields, from materials science and engineering to geology and chemistry. This article delves deep into the factors determining the formation of crystalline solids, exploring various material categories and their propensity to exhibit crystalline structures.
The Fundamentals of Crystalline Solids
Before we delve into specific materials, let's establish a firm grasp on what defines a crystalline solid. The key characteristic is the long-range order of its constituent atoms, ions, or molecules. This order manifests as a repeating three-dimensional pattern called a unit cell. This unit cell, the smallest repeating unit, is replicated throughout the entire crystal lattice, creating the macroscopic crystalline structure. The regularity of this arrangement influences many of the material's physical properties, including its melting point, hardness, cleavage planes, and optical properties. In contrast, amorphous solids, like glass, lack this long-range order, resulting in different properties.
Factors Influencing Crystalline Solid Formation
Several factors play pivotal roles in determining whether a material will form a crystalline solid:
-
Chemical Bonding: The type of chemical bonding present significantly influences the likelihood of crystalline structure formation. Ionic bonds, characterized by electrostatic attraction between oppositely charged ions (e.g., NaCl), often lead to highly ordered crystalline structures due to the strong and directional nature of the interaction. Covalent bonds, involving shared electron pairs (e.g., diamond), can also result in crystalline solids, although the complexity of the bonding network can sometimes lead to amorphous structures. Metallic bonds, found in metals, involve a "sea" of delocalized electrons, and readily facilitate crystalline structures because of the non-directional nature of the bonding. Van der Waals forces and hydrogen bonds, though weaker, can still contribute to crystalline structures in certain molecules, particularly at lower temperatures.
-
Cooling Rate: The rate at which a liquid or melt cools drastically affects the final solid state. Slow cooling allows atoms or molecules sufficient time to arrange themselves into an ordered crystalline structure. Rapid cooling, however, often prevents this ordering, leading to the formation of an amorphous solid. This is why volcanic glass (obsidian) is amorphous; the lava cools too quickly for crystallization to occur.
-
Impurities: The presence of impurities in a material can disrupt the regular arrangement of atoms, hindering the formation of a perfectly crystalline structure. Impurities can act as nucleation sites, affecting crystal growth and leading to defects within the crystal lattice. While complete purity is rare, the concentration of impurities plays a critical role.
-
Pressure: Pressure influences the density of a material, affecting the interatomic distances and consequently the energetics of crystallization. High pressures can favor specific crystal structures that might not be stable at lower pressures. This is particularly relevant in geological settings, where high pressures contribute to the formation of polymorphs—minerals with the same chemical composition but different crystal structures.
Material Categories and Crystalline Structures
Now, let's examine various materials and their likelihood of forming crystalline solids:
1. Metals
Most metals readily form crystalline solids. Their metallic bonding, with delocalized electrons, allows for flexible and relatively strong bonding in multiple directions, facilitating the formation of well-ordered structures like body-centered cubic (BCC), face-centered cubic (FCC), and hexagonal close-packed (HCP) arrangements. The specific crystal structure adopted depends on factors like atomic size and electronic configuration. Examples include iron (BCC), aluminum (FCC), and zinc (HCP).
2. Ionic Compounds
Ionic compounds, formed by electrostatic attraction between cations and anions, frequently exhibit crystalline structures. The strong Coulombic forces between ions lead to well-defined and highly ordered lattices. The crystal structure is influenced by the size and charge ratio of the ions. NaCl (table salt), with its simple cubic structure, exemplifies this behavior. Other examples include MgO (magnesium oxide) and CaF₂ (calcium fluoride), each with its characteristic crystal structure.
3. Covalent Network Solids
Covalent network solids, characterized by a continuous network of covalent bonds throughout the material, often form crystalline solids. The strong directional nature of the covalent bonds dictates the arrangement of atoms, leading to well-defined crystal structures. Diamond, with its tetrahedral arrangement of carbon atoms, is a prime example. Silicon and germanium, also group 14 elements, exhibit similar diamond-like structures. However, the complexity of the bonding can sometimes lead to amorphous structures, particularly if the cooling rate is rapid.
4. Molecular Solids
Molecular solids are composed of discrete molecules held together by relatively weak intermolecular forces like van der Waals forces or hydrogen bonds. The ability to form a crystalline solid depends heavily on the shape and polarity of the molecules. Symmetrical molecules with strong intermolecular interactions are more likely to crystallize, while irregular, non-polar molecules may form amorphous solids. Examples of molecular solids that form crystalline structures include ice (H₂O), solid CO₂, and many organic compounds. The crystalline structure reflects the molecular packing efficiency, maximizing intermolecular attractions while minimizing repulsions.
5. Polymers
Polymers, consisting of long chains of repeating units (monomers), can exhibit both crystalline and amorphous regions. The degree of crystallinity depends on factors such as polymer chain regularity, chain flexibility, and intermolecular interactions. Highly regular polymers with strong intermolecular forces are more likely to form crystalline regions. Polyethylene, for example, can exhibit crystallinity due to the regular arrangement of its carbon chain. However, many polymers are predominantly amorphous due to chain irregularities, branching, and weaker intermolecular forces.
6. Ceramics
Ceramics, generally inorganic, non-metallic solids, often form crystalline structures. They are typically composed of ionic and/or covalent bonds. The specific crystal structure depends on the chemical composition and the processing conditions during manufacturing. Examples include alumina (Al₂O₃), zirconia (ZrO₂), and silicon carbide (SiC), all having characteristic crystalline structures that influence their mechanical, electrical, and thermal properties.
Amorphous Solids: A Contrast
It's important to distinguish crystalline solids from amorphous solids. Amorphous solids lack the long-range order characteristic of crystalline materials. Their atomic arrangement is disordered and random. While many materials can form both crystalline and amorphous structures depending on processing conditions, some materials are inherently more prone to forming amorphous structures. Examples of amorphous materials include glass, certain polymers, and some alloys. Their lack of long-range order significantly affects their properties, often resulting in lower strength, greater brittleness, and lower melting points compared to their crystalline counterparts.
Techniques for Analyzing Crystalline Structures
Several techniques are used to characterize the crystalline structure of materials:
-
X-ray diffraction (XRD): XRD is a powerful technique that uses the diffraction of X-rays by the crystal lattice to determine the arrangement of atoms within the material. The diffraction pattern provides information about the unit cell dimensions, symmetry, and the arrangement of atoms within the unit cell.
-
Electron diffraction: Similar to XRD, electron diffraction uses electron beams to probe the crystal structure. It is particularly useful for analyzing thin films and nanomaterials.
-
Neutron diffraction: Neutron diffraction utilizes neutron beams to study crystal structures. It's especially valuable for determining the positions of light atoms in the presence of heavy atoms, something that can be challenging with XRD.
Conclusion
The formation of crystalline solids is a complex phenomenon influenced by several interacting factors. Understanding these factors—chemical bonding, cooling rate, impurities, and pressure—is crucial for predicting and controlling the crystalline structure of materials. This knowledge is essential across various disciplines, enabling the design and development of materials with tailored properties for specific applications. From the shimmering facets of a diamond to the robust strength of a metal alloy, the orderly arrangement of atoms in crystalline solids underpins much of the material world around us. The ongoing research into crystalline solids continues to unveil new insights into the relationship between structure and properties, driving innovation in materials science and technology.
Latest Posts
Latest Posts
-
Stacking And Piling Is Another Term For What Structural System
May 11, 2025
-
Which Action Most Makes Creon A Villain In This Story
May 11, 2025
-
Match Each Graph With Its Table
May 11, 2025
-
Comparison Of Crayfish And Grasshopper Table
May 11, 2025
-
Hershey Trust Has Equity In The Corporation Which Means
May 11, 2025
Related Post
Thank you for visiting our website which covers about Which Of The Following Materials May Form Crystalline Solids . We hope the information provided has been useful to you. Feel free to contact us if you have any questions or need further assistance. See you next time and don't miss to bookmark.