Why Is The Entropy Change Negative For Ring Closures
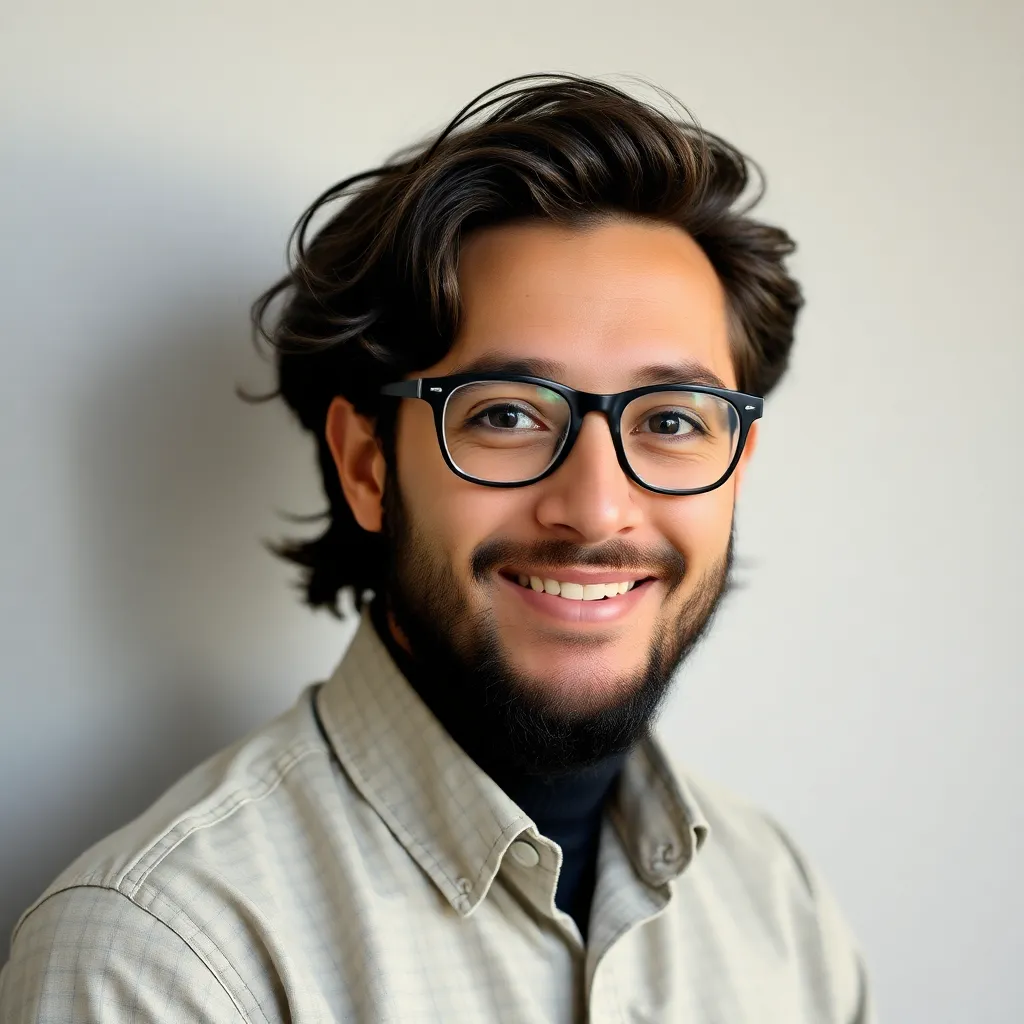
Onlines
May 05, 2025 · 6 min read

Table of Contents
Why is the Entropy Change Negative for Ring Closures?
Ring closure reactions, a fundamental class of transformations in organic chemistry, often exhibit a surprising characteristic: a negative entropy change (ΔS). This seemingly counterintuitive result, where the system becomes more ordered, stands in contrast to many other reactions where the increased randomness leads to a positive ΔS. Understanding why this happens requires a deep dive into the underlying principles of thermodynamics and statistical mechanics. This article will explore the reasons behind the negative entropy change in ring closure reactions, examining the factors that contribute to this phenomenon and providing concrete examples.
The Entropy Concept: A Recap
Before delving into the specifics of ring closure, let's briefly revisit the concept of entropy. Entropy (S) is a thermodynamic state function that measures the randomness or disorder of a system. A system with high entropy is highly disordered, while a system with low entropy is highly ordered. The second law of thermodynamics dictates that the total entropy of an isolated system can only increase over time or remain constant in ideal cases where the system is in a steady state or undergoing a reversible process. In simpler terms, things tend towards chaos.
The change in entropy (ΔS) during a reaction is a crucial factor in determining its spontaneity. A positive ΔS favors the reaction proceeding spontaneously, while a negative ΔS opposes it. The Gibbs free energy equation (ΔG = ΔH - TΔS) elegantly combines enthalpy (ΔH) and entropy changes to predict spontaneity, where T represents the temperature.
Why Ring Closure Reactions Often Have Negative ΔS
The negative entropy change associated with ring closure reactions stems primarily from the loss of translational and rotational freedom experienced by the reacting molecules. Let's break this down:
1. Loss of Translational Freedom
Before ring closure, the two reacting ends of a molecule are relatively free to move independently in three-dimensional space. These independent movements contribute significantly to the overall entropy of the system. Upon ring formation, these two ends become tethered together, significantly restricting their translational freedom. This restriction translates directly to a decrease in the number of accessible microstates – the number of possible arrangements of molecules at a given energy – and, consequently, a decrease in entropy.
Imagine two independently moving dancers. Their movement across the dance floor contributes significantly to the overall randomness of the dance scene. Once they join hands, forming a couple, their movements become far more restricted, reducing the randomness of the scene. This analogy beautifully illustrates the loss of translational freedom during ring closure.
2. Loss of Rotational Freedom
Similar to translational freedom, the reacting ends of a molecule also possess rotational freedom before ring closure. Each end can rotate independently around its bonds. Once the ring forms, this rotational freedom is severely restricted, or in some cases, completely eliminated. This further contributes to the decrease in the number of accessible microstates and hence, to a negative ΔS.
Consider the two dancers again. Before joining hands, each can spin independently. Once coupled, their rotational freedom becomes coupled and restricted to the synchronized movements of the pair. This mirrors the decrease in rotational freedom associated with ring closure.
3. The Size of the Ring Matters
The magnitude of the negative entropy change in ring closure is strongly influenced by the size of the ring being formed. Smaller rings (three- to five-membered) experience a more significant reduction in entropy compared to larger rings (six-membered and above). This is because smaller rings are inherently more rigid and constrained, resulting in a greater loss of freedom.
Smaller rings face a greater degree of ring strain, forcing the atoms into less favorable geometries. This increased strain further reduces the conformational flexibility and hence contributes to the negative entropy. Larger rings have more conformational flexibility, offsetting to some extent the entropy decrease associated with ring closure.
4. Solvent Effects
The solvent in which the reaction takes place can also influence the entropy change. A highly ordered solvent, for example, might restrict the mobility of the reacting molecules, leading to a smaller decrease in entropy upon ring closure. Conversely, a more disordered solvent might allow for greater mobility, making the decrease in entropy upon ring closure more pronounced.
5. Steric Effects
Steric hindrance, or the repulsion between atoms or groups of atoms in close proximity, can also play a role in the entropy change. If the reacting groups are bulky, the ring closure might be less favored due to steric strain, further influencing the negative entropy change. This steric strain restricts the possible conformations which adds to the decrease in entropy.
Illustrative Examples
Let's consider some examples to highlight the negative entropy change in ring closure:
-
Lactone formation: The formation of a cyclic ester (lactone) from a hydroxy acid involves the intramolecular reaction between the hydroxyl and carboxyl groups. This reaction often has a negative entropy change due to the loss of translational and rotational freedom upon ring formation. The smaller the ring, the more negative the entropy change.
-
Lactam formation: Similarly, the formation of a cyclic amide (lactam) from an amino acid displays a negative entropy change. Again, the size of the ring impacts the magnitude of the negative ΔS.
-
Intramolecular aldol condensation: The intramolecular aldol condensation involves the reaction between two carbonyl groups within the same molecule. The formation of the cyclic product leads to a significant reduction in entropy.
Overcoming the Negative Entropy Change
Despite the unfavorable entropy change, ring closure reactions can still proceed spontaneously if the enthalpy change (ΔH) is sufficiently negative. A highly exothermic reaction (large negative ΔH) can overcome the unfavorable entropy term, making the overall Gibbs free energy change negative (ΔG < 0) and rendering the reaction spontaneous. This often occurs when strong bonds are formed in the ring closure process. For example, the formation of a strong ester bond in lactone formation can drive the reaction forward despite the negative entropy.
Conclusion
The negative entropy change in ring closure reactions is a consequence of the restricted movement of the reacting molecules upon ring formation. This restriction manifests in the loss of translational and rotational freedom, leading to a decrease in the number of accessible microstates and hence, a negative ΔS. While the negative entropy term opposes spontaneity, a sufficiently exothermic reaction can overcome this unfavorable contribution, making the overall process spontaneous. The size of the ring, solvent effects, steric factors, and the nature of the newly formed bonds all play crucial roles in determining the magnitude of the entropy change and ultimately the spontaneity of the ring closure reaction. Understanding these factors is critical for predicting and controlling the outcome of such reactions in synthetic chemistry.
Latest Posts
Latest Posts
-
If Lk Mk Lk 7x 10
May 05, 2025
-
Effective Target Width Is A Measure Of
May 05, 2025
-
Which Of The Following Accurately Describes Circuits
May 05, 2025
-
Chapter 10 Summary Roll Of Thunder Hear My Cry
May 05, 2025
-
A Symphony Orchestra Is An Example Of A Chamber Ensemble
May 05, 2025
Related Post
Thank you for visiting our website which covers about Why Is The Entropy Change Negative For Ring Closures . We hope the information provided has been useful to you. Feel free to contact us if you have any questions or need further assistance. See you next time and don't miss to bookmark.