Experiment 5 The Importance Of Cell Cycle Control
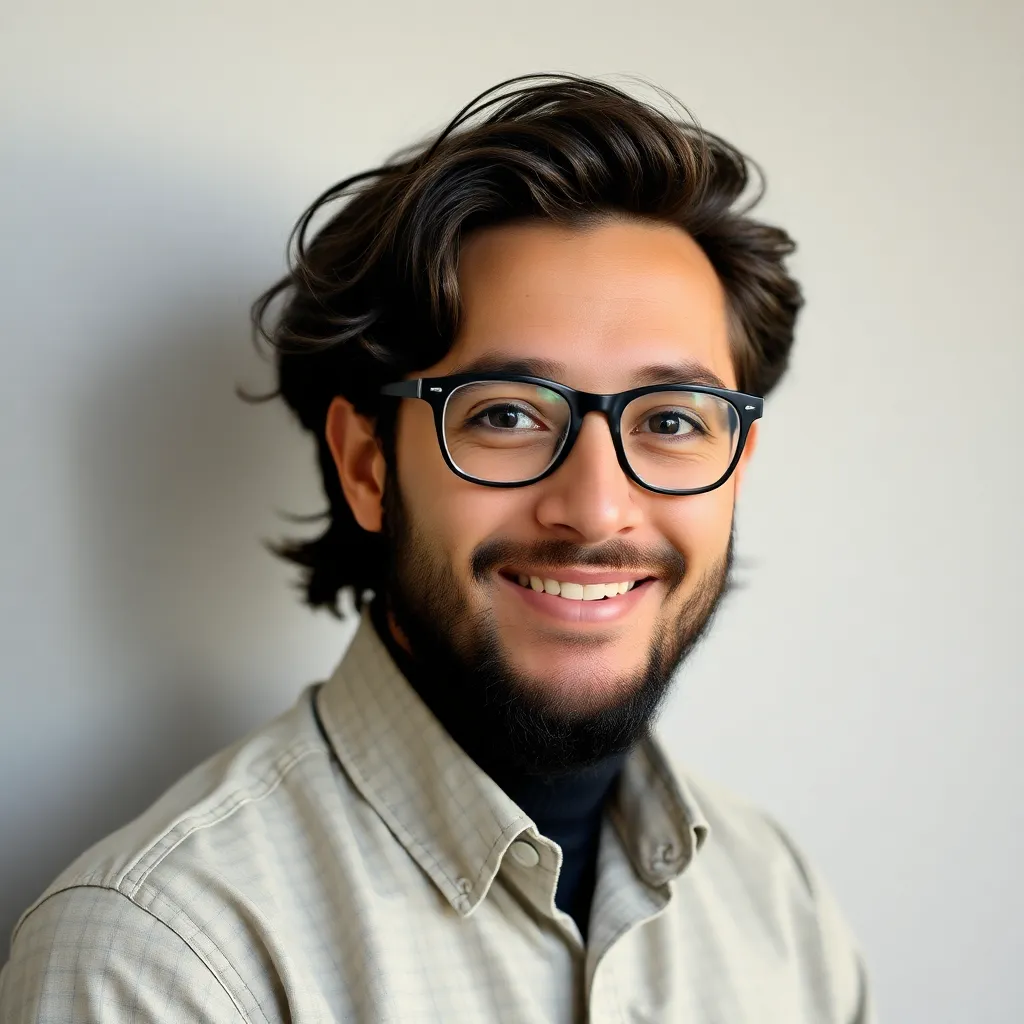
Onlines
Apr 07, 2025 · 6 min read
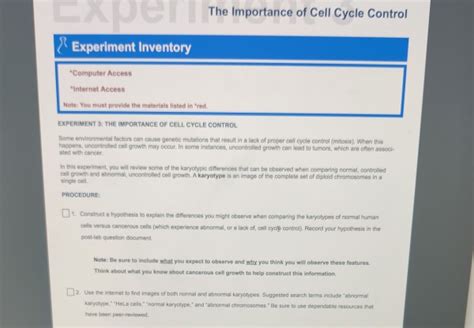
Table of Contents
Experiment 5: The Importance of Cell Cycle Control
The cell cycle, a tightly regulated series of events leading to cell growth and division, is fundamental to life. Its precise control is crucial for the development, maintenance, and repair of multicellular organisms. Dysregulation of the cell cycle can lead to catastrophic consequences, including uncontrolled cell growth – a hallmark of cancer. This experiment delves into the vital importance of cell cycle control mechanisms, exploring the consequences of their disruption.
Understanding the Cell Cycle
Before examining the consequences of cell cycle deregulation, it's essential to grasp the basic stages:
1. Interphase:
- G1 (Gap 1): The cell grows in size, produces RNA and synthesizes proteins needed for DNA replication. This is a crucial checkpoint, assessing cell size and DNA integrity before committing to replication.
- S (Synthesis): DNA replication occurs, creating two identical copies of each chromosome. Careful regulation prevents errors during this critical stage.
- G2 (Gap 2): The cell continues to grow and prepares for mitosis. Another checkpoint ensures DNA replication was successful and the cell is ready to divide.
2. M Phase (Mitosis):
- Prophase: Chromosomes condense and become visible, the nuclear envelope breaks down, and the mitotic spindle forms.
- Metaphase: Chromosomes align at the metaphase plate, ensuring equal segregation to daughter cells. This is a crucial checkpoint, preventing premature separation of chromosomes.
- Anaphase: Sister chromatids separate and move to opposite poles of the cell.
- Telophase: Chromosomes decondense, the nuclear envelope reforms, and the cell begins to divide.
- Cytokinesis: The cytoplasm divides, resulting in two daughter cells, each with a complete set of chromosomes.
Cell Cycle Checkpoints: Guardians of Genomic Integrity
The cell cycle isn't a linear process; it's punctuated by checkpoints that monitor the cell's internal state and external environment. These checkpoints ensure that each step is completed accurately before proceeding to the next. The major checkpoints are:
-
G1 Checkpoint: This is the most important checkpoint, deciding whether the cell will proceed to DNA replication. It assesses cell size, nutrient availability, growth factors, and DNA damage. If conditions are unfavorable or DNA is damaged, the cell cycle is arrested, allowing for repair or programmed cell death (apoptosis).
-
G2 Checkpoint: This checkpoint checks for successful DNA replication and DNA damage. If errors are detected, the cell cycle is halted, providing time for repair before mitosis.
-
M Checkpoint (Spindle Checkpoint): This checkpoint ensures that all chromosomes are properly attached to the mitotic spindle before anaphase begins. This prevents aneuploidy (abnormal chromosome number), a common feature of cancer cells.
The Consequences of Cell Cycle Dysregulation
When the cell cycle control mechanisms fail, the consequences can be severe. Uncontrolled cell division leads to the formation of tumors, a defining characteristic of cancer. Several factors contribute to this dysregulation:
1. Mutations in Cell Cycle Genes:
Genes encoding proteins that regulate the cell cycle are frequent targets of mutations. These mutations can lead to:
-
Loss of function: Mutations in tumor suppressor genes like p53 and Rb (retinoblastoma protein) eliminate their ability to inhibit cell cycle progression, leading to uncontrolled growth. p53, often called the "guardian of the genome," plays a critical role in detecting DNA damage and initiating apoptosis if the damage is irreparable. Rb acts as a brake on cell cycle progression, preventing entry into S phase unless conditions are favorable.
-
Gain of function: Mutations in proto-oncogenes, which normally promote cell cycle progression, can convert them into oncogenes, driving uncontrolled cell division. Examples include Ras and Myc, which promote cell growth and division. Oncogene activation can bypass normal cell cycle checkpoints, resulting in continuous proliferation.
2. Telomere Shortening and Dysfunction:
Telomeres, protective caps at the ends of chromosomes, shorten with each cell division. Critically short telomeres can trigger cell cycle arrest or apoptosis. However, cancer cells often express telomerase, an enzyme that maintains telomere length, enabling them to bypass this natural growth restriction.
3. External Factors:
Environmental factors can also contribute to cell cycle dysregulation. Exposure to carcinogens, such as radiation and certain chemicals, can damage DNA, leading to mutations in cell cycle regulatory genes and causing uncontrolled cell division. Chronic inflammation and hormonal imbalances can also play a role.
Experiment Design: Investigating Cell Cycle Control
A well-designed experiment can provide invaluable insights into the mechanisms of cell cycle control and the consequences of its disruption. Here's a possible experimental setup:
Hypothesis: Disruption of cell cycle checkpoints leads to increased cell proliferation and genomic instability.
Materials:
- Cell culture system (e.g., human fibroblasts)
- Cell cycle inhibitors (e.g., inhibitors of cyclin-dependent kinases (CDKs))
- DNA damaging agents (e.g., UV radiation, alkylating agents)
- Fluorescent cell cycle dyes (e.g., propidium iodide)
- Flow cytometry equipment
- Microscopy equipment
- Methods for assessing genomic instability (e.g., chromosome spreads, micronucleus assay)
Procedure:
-
Cell Culture: Establish a cell culture of human fibroblasts.
-
Treatment Groups: Divide the cells into several treatment groups:
- Control group (untreated)
- Cell cycle inhibitor treatment group (e.g., exposure to a CDK inhibitor)
- DNA damaging agent treatment group (e.g., UV irradiation)
- Combination treatment group (CDK inhibitor + DNA damaging agent)
-
Incubation: Incubate the cells for a specific time period (e.g., 24, 48, 72 hours).
-
Cell Cycle Analysis:
- Harvest cells from each treatment group.
- Stain cells with a fluorescent cell cycle dye (e.g., propidium iodide).
- Analyze cell cycle distribution using flow cytometry. This will determine the proportion of cells in each phase of the cell cycle (G1, S, G2/M).
-
Genomic Instability Assessment:
- Use appropriate methods (e.g., chromosome spreads, micronucleus assay) to assess the level of genomic instability in each treatment group.
-
Data Analysis:
- Analyze the flow cytometry data to determine the effect of treatments on cell cycle progression. Compare the cell cycle profiles of treated cells with the control group.
- Quantify genomic instability in each treatment group and compare it to the control group.
Expected Results:
- The control group should show a normal cell cycle distribution.
- Treatment with cell cycle inhibitors should increase the proportion of cells arrested in a specific phase of the cell cycle, depending on the target of the inhibitor.
- Treatment with DNA damaging agents should lead to increased cell cycle arrest and potentially apoptosis.
- The combination treatment may exhibit a synergistic effect, leading to a greater degree of cell cycle arrest and/or apoptosis.
- Increased genomic instability (e.g., increased micronuclei formation) should be observed in the groups treated with DNA damaging agents.
Conclusion:
This experiment will demonstrate the critical role of cell cycle checkpoints in maintaining genomic integrity and preventing uncontrolled cell proliferation. By disrupting these checkpoints, it is possible to observe the consequences – increased cell proliferation and genomic instability – which provides insights into the mechanisms that underlie cancer development.
Implications for Cancer Therapy
Understanding cell cycle control is vital for developing effective cancer therapies. Many anticancer drugs target cell cycle machinery, aiming to selectively kill cancer cells by disrupting their uncontrolled growth. Examples include:
-
CDK inhibitors: These drugs block the activity of CDKs, halting cell cycle progression.
-
Topoisomerase inhibitors: These drugs interfere with DNA replication and repair, leading to cell death.
-
Microtubule inhibitors: These drugs disrupt microtubule dynamics, preventing proper chromosome segregation during mitosis.
These drugs highlight the therapeutic potential of targeting cell cycle regulation in cancer treatment. Future research focused on understanding the complex interplay of cell cycle control mechanisms will likely lead to the development of more effective and targeted cancer therapies. The ability to precisely manipulate cell cycle checkpoints could revolutionize cancer treatment, potentially minimizing side effects while maximizing therapeutic efficacy. Further exploration of specific pathways and their interactions, coupled with advanced experimental techniques, will undoubtedly uncover even more nuances in this critically important area of biological research. This continuous pursuit of knowledge is essential in the fight against cancer and in maintaining a deeper understanding of the fundamental processes of life.
Latest Posts
Latest Posts
-
9 2 6 Secure A Home Wireless Network
Apr 07, 2025
-
Energy Skate Park App1 Lab 1 Answer Key
Apr 07, 2025
-
Behavioral Therapy For Self Mutilation Ati
Apr 07, 2025
-
10 5 Additional Practice Secant Lines And Segments
Apr 07, 2025
-
For External Reporting Income Statements Are Generally Prepared Using
Apr 07, 2025
Related Post
Thank you for visiting our website which covers about Experiment 5 The Importance Of Cell Cycle Control . We hope the information provided has been useful to you. Feel free to contact us if you have any questions or need further assistance. See you next time and don't miss to bookmark.