The Cellular Basis For Bacterial Resistance To Antimicrobics Include
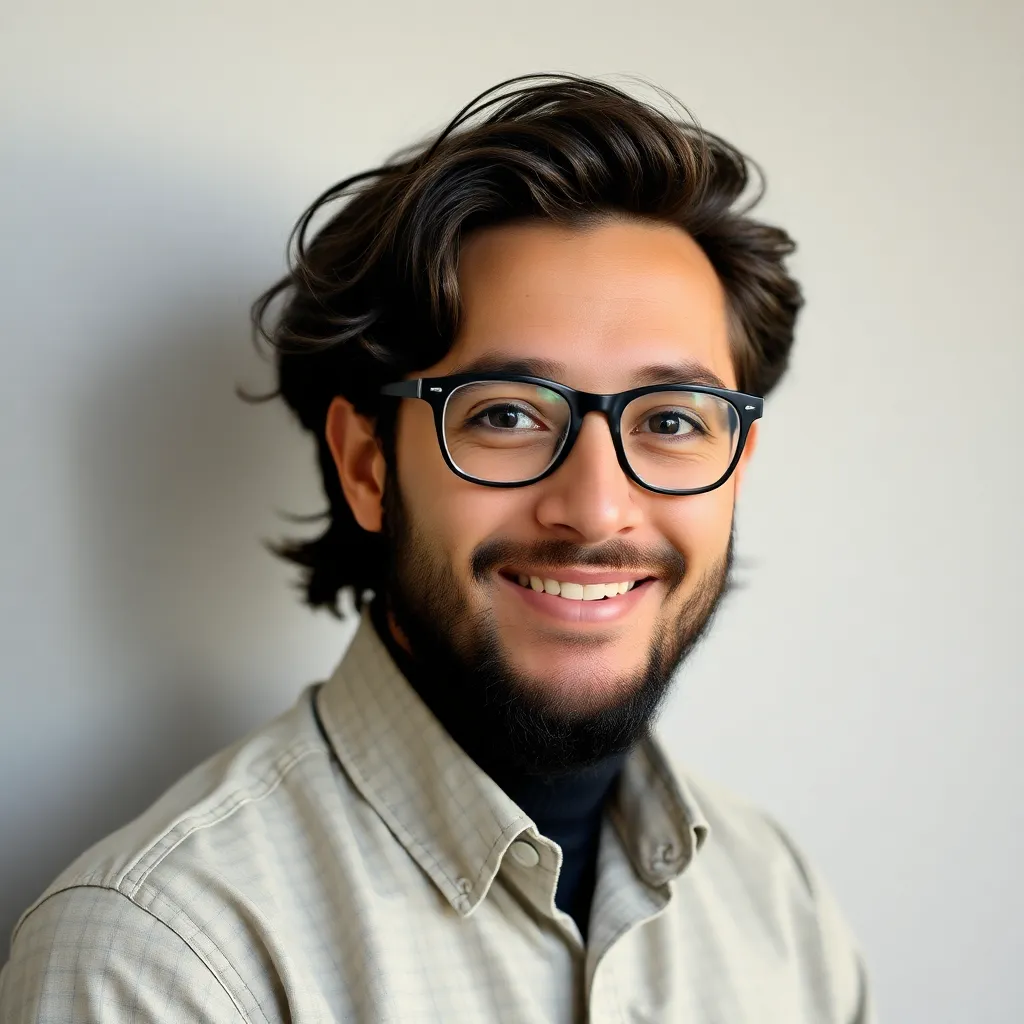
Onlines
Apr 24, 2025 · 6 min read

Table of Contents
The Cellular Basis for Bacterial Resistance to Antimicrobics
Antimicrobial resistance (AMR) poses a significant threat to global health, jeopardizing the effectiveness of treatments for bacterial infections. Understanding the cellular mechanisms underlying this resistance is crucial for developing new strategies to combat it. This article delves into the diverse cellular processes and adaptations that bacteria employ to withstand the effects of antimicrobics.
Mechanisms of Antimicrobial Resistance
Bacterial resistance to antimicrobics arises from a variety of mechanisms, often involving multiple factors working in concert. These mechanisms can be broadly classified into several categories:
1. Target Modification
Many antimicrobics exert their effects by targeting specific cellular components or processes within bacteria. Resistance can develop through alterations in the structure or function of these targets, rendering them less susceptible to the drug.
-
Mutation in Target Genes: Point mutations or larger-scale genetic changes in the genes encoding antimicrobial targets can alter the binding site of the drug, reducing its affinity and effectiveness. For example, mutations in the gyrA and parC genes, encoding subunits of DNA gyrase and topoisomerase IV, respectively, are commonly associated with fluoroquinolone resistance. These mutations can significantly alter the drug binding site.
-
Target Overexpression: Increasing the production of the target molecule can overwhelm the antimicrobial's capacity to inhibit its function. For instance, increased production of dihydrofolate reductase (DHFR), the target of trimethoprim, can lead to resistance. The sheer abundance of the target reduces the drug's impact.
-
Target Masking: Certain bacteria can modify their target molecules by adding chemical groups, such as methylations, that interfere with antimicrobial binding. This is a key mechanism of resistance to aminoglycosides, where enzymatic modifications to ribosomal RNA prevent antibiotic binding.
2. Efflux Pumps
Bacteria possess efflux pumps, transmembrane proteins that actively expel various molecules, including antimicrobics, from the cytoplasm. Overexpression or modification of these pumps can significantly reduce intracellular drug concentrations, leading to resistance.
-
Increased Expression: Stressful conditions, including antimicrobial exposure, can trigger the upregulation of efflux pump genes, leading to increased efflux activity. This is a common mechanism of multidrug resistance, where a single pump can expel multiple classes of antimicrobics. The MexAB-OprM efflux pump in Pseudomonas aeruginosa is a prime example.
-
Efflux Pump Mutation: Mutations in the genes encoding efflux pumps can enhance their activity or broaden their substrate specificity, leading to increased resistance to a wider range of antimicrobics. These mutations can affect pump substrate binding or the energy-coupling mechanism.
3. Enzyme Inactivation
Bacteria can produce enzymes that chemically modify or degrade antimicrobics, rendering them inactive. This is a crucial mechanism of resistance, particularly for β-lactam antibiotics.
-
β-Lactamase Production: Many bacteria produce β-lactamases, enzymes that hydrolyze the β-lactam ring of penicillin, cephalosporin, and other β-lactam antibiotics, inactivating them. Different types of β-lactamases exhibit varying substrate specificities and resistance profiles. Extended-spectrum β-lactamases (ESBLs) and carbapenemases pose major clinical challenges.
-
Aminoglycoside-Modifying Enzymes: Bacteria can produce enzymes that modify aminoglycosides through processes such as acetylation, adenylation, or phosphorylation, reducing their binding affinity to the ribosome. These modifications effectively neutralize the antibiotic's effect.
4. Altered Permeability
The bacterial cell envelope, comprising the cell wall and cytoplasmic membrane, acts as a permeability barrier to many antimicrobics. Modifications in the structure or composition of this barrier can reduce drug uptake, leading to resistance.
-
Cell Wall Alterations: Changes in the cell wall structure, such as reduced peptidoglycan cross-linking or altered porin composition, can hinder the penetration of antimicrobics. This mechanism is especially relevant for β-lactams and other cell-wall-targeting agents.
-
Membrane Modifications: Alterations in the lipid composition of the cytoplasmic membrane can influence drug permeability. For example, changes in lipopolysaccharide (LPS) structure in Gram-negative bacteria can affect the entry of certain antimicrobics.
Genetic Basis of Resistance
The genetic basis of antimicrobial resistance is multifaceted, encompassing various mechanisms of genetic exchange and mutation.
1. Chromosomal Mutations
Spontaneous mutations in chromosomal genes encoding antimicrobial targets or efflux pumps can confer resistance. These mutations arise randomly through errors during DNA replication. Selection pressure from antimicrobial exposure favors the survival and proliferation of resistant mutants.
2. Horizontal Gene Transfer
Horizontal gene transfer (HGT) plays a crucial role in the rapid spread of resistance genes among bacterial populations. HGT mechanisms include:
-
Conjugation: Direct transfer of genetic material between bacteria through a pilus. Plasmids carrying resistance genes are frequently transferred through this mechanism.
-
Transformation: Uptake of free DNA from the environment. Bacteria can incorporate resistance genes from lysed cells.
-
Transduction: Transfer of genetic material through bacteriophages (viruses that infect bacteria). Phage-mediated transfer can move resistance genes between bacterial populations.
3. Mobile Genetic Elements
Resistance genes are often located on mobile genetic elements, such as plasmids and transposons. These elements can move freely within and between bacterial genomes, facilitating the rapid spread of resistance.
-
Plasmids: Circular DNA molecules that replicate independently of the bacterial chromosome. Plasmids often carry multiple resistance genes, contributing to multidrug resistance.
-
Transposons: "Jumping genes" that can move from one location in the genome to another. Transposons can carry resistance genes and integrate them into different genetic contexts.
-
Integrons: Genetic elements that capture and express gene cassettes, often containing resistance genes. Integrons facilitate the acquisition and dissemination of multiple resistance genes.
Clinical Implications and Future Perspectives
The widespread emergence of antimicrobial resistance poses a serious threat to global health. Infections caused by resistant bacteria are more difficult and expensive to treat, leading to increased morbidity, mortality, and healthcare costs. The development of new antimicrobics and strategies to combat AMR is of paramount importance.
Strategies to Combat AMR
Combating AMR requires a multi-pronged approach, including:
-
Development of new antibiotics: Research and development efforts are crucial to discover and develop new antimicrobics targeting novel cellular pathways.
-
Optimization of antibiotic stewardship: Implementing strategies to optimize the use of existing antibiotics, including preventing overuse and misuse.
-
Development of alternative therapies: Exploring alternative treatments such as bacteriophages, immunotherapies, and vaccines.
-
Combating the spread of resistance: Implementing infection control measures to prevent the spread of resistant bacteria in healthcare settings and the community.
-
Addressing the misuse of antibiotics in agriculture: Reducing the use of antibiotics in animal agriculture to minimize the selection pressure that promotes resistance development.
Conclusion
The cellular basis of bacterial resistance to antimicrobics is complex and multifaceted. Understanding the diverse mechanisms of resistance, including target modification, efflux pump activity, enzyme inactivation, and altered permeability, is crucial for developing effective strategies to combat this growing global threat. A multidisciplinary approach combining research, clinical practice, and public health initiatives is essential to mitigate the impact of antimicrobial resistance and safeguard public health. The continuous evolution of bacterial resistance necessitates ongoing research and innovation to maintain effective control over bacterial infections. Improved diagnostics, novel therapeutic approaches, and responsible antibiotic stewardship are key to navigating this evolving challenge in the fight against infectious diseases.
Latest Posts
Latest Posts
-
Escoge Los Pronombres Correctos Para Completar Las Oraciones
Apr 24, 2025
-
Martha Is Considering Acquiring Another Piano
Apr 24, 2025
-
3 12 Unit Test Polynomials Part 1
Apr 24, 2025
-
Why Might Some Ecoregions Have More Species Than Others
Apr 24, 2025
-
The Catcher In The Rye Chapter 24
Apr 24, 2025
Related Post
Thank you for visiting our website which covers about The Cellular Basis For Bacterial Resistance To Antimicrobics Include . We hope the information provided has been useful to you. Feel free to contact us if you have any questions or need further assistance. See you next time and don't miss to bookmark.