This Neuron Is Most Depolarized At Mv
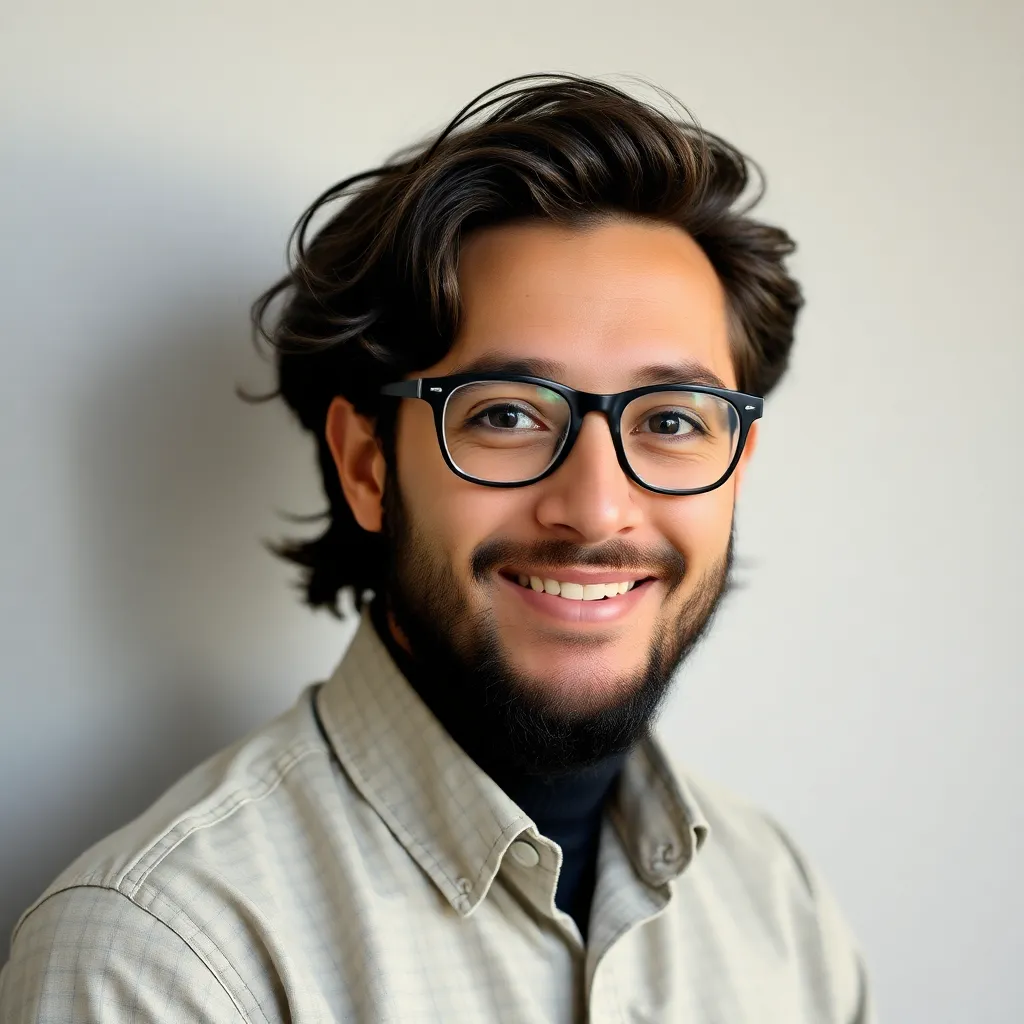
Onlines
May 05, 2025 · 6 min read

Table of Contents
This Neuron is Most Depolarized at mV: Understanding Membrane Potential and Neuronal Excitability
The question "This neuron is most depolarized at mV" delves into the fascinating world of neuronal electrophysiology. Understanding membrane potential and its fluctuations is crucial for comprehending how neurons communicate and function. This article will explore the concept of depolarization, the factors influencing membrane potential, and the significance of the specific voltage at which a neuron reaches its peak depolarization.
What is Membrane Potential?
The membrane potential is the difference in electrical potential between the inside and the outside of a neuron's cell membrane. This difference arises from an uneven distribution of ions, primarily sodium (Na+), potassium (K+), chloride (Cl-), and calcium (Ca2+), across the membrane. At rest, the inside of the neuron is typically more negative than the outside, a state known as the resting membrane potential. This resting potential, usually around -70 mV, is maintained by various ion channels and pumps embedded within the cell membrane.
The Role of Ion Channels and Pumps
Several key players maintain the resting membrane potential:
-
Potassium Leak Channels: These channels allow potassium ions to passively diffuse out of the cell down their concentration gradient. This outward movement of positive charge contributes to the negative interior of the cell.
-
Sodium-Potassium Pump (Na+/K+ ATPase): This active transport mechanism actively pumps three sodium ions out of the cell and two potassium ions into the cell for every molecule of ATP it uses. This further contributes to the negative membrane potential by removing more positive charges (Na+) than it brings in (K+).
-
Other Ion Channels: While potassium leak channels are dominant in establishing the resting potential, other ion channels, such as chloride channels and sodium channels, play smaller, yet crucial, roles in fine-tuning the membrane potential and influencing its responsiveness to stimuli.
Depolarization: A Shift Towards Excitation
Depolarization refers to a decrease in the magnitude of the membrane potential. In simpler terms, it's a process where the inside of the neuron becomes less negative, moving closer to zero mV. This shift is crucial for neuronal signaling as it brings the membrane potential closer to the threshold potential, the voltage needed to trigger an action potential.
The Process of Depolarization
Depolarization is typically initiated by the influx of positive ions, predominantly sodium ions (Na+), into the neuron. This influx can be triggered by various stimuli, including:
-
Neurotransmitters: Chemical messengers released from other neurons bind to receptors on the postsynaptic membrane, opening ligand-gated ion channels, often allowing Na+ to enter the cell.
-
Sensory Stimuli: Physical stimuli, such as light, sound, or pressure, can activate sensory neurons, opening ion channels and initiating depolarization.
-
Electrical Stimuli: Direct electrical stimulation can also depolarize a neuron by artificially increasing the membrane potential.
The All-or-None Principle of Action Potentials
Once the membrane potential reaches the threshold potential (typically around -55 mV, although this varies between neuron types), a rapid and dramatic change occurs: an action potential. The action potential is an all-or-none event; it either happens completely or not at all. This means that if the threshold is reached, a full-fledged action potential will propagate down the axon, regardless of the intensity of the initial depolarization above the threshold.
The Significance of the Peak Depolarization Voltage
The specific mV at which a neuron reaches its peak depolarization is not a fixed value. It varies depending on various factors, including:
-
Neuron Type: Different types of neurons have different ion channel compositions and thus different sensitivities to depolarizing stimuli. This leads to variations in the peak depolarization voltage.
-
Stimulus Intensity: A stronger stimulus will generally lead to a more significant depolarization, resulting in a higher peak voltage before the repolarization phase begins.
-
Ion Channel Properties: The kinetics and conductance of various ion channels, including the activation and inactivation times of voltage-gated sodium and potassium channels, significantly influence the shape and peak voltage of the action potential.
-
Temperature: Temperature influences the kinetics of ion channels, affecting the speed and magnitude of depolarization and repolarization.
Repolarization and Hyperpolarization
After reaching its peak depolarization, the neuron undergoes repolarization, a process where the membrane potential returns towards its resting value. This is largely due to the closure of voltage-gated sodium channels and the opening of voltage-gated potassium channels. Potassium ions flow out of the cell, restoring the negative membrane potential. In some cases, the membrane potential can briefly become even more negative than the resting potential, a state called hyperpolarization. Hyperpolarization represents a period of reduced excitability, making the neuron less likely to fire another action potential immediately.
Factors Affecting Membrane Potential Beyond Basic Ion Dynamics
Beyond the fundamental roles of ion channels and pumps, several other factors contribute to the complexity of neuronal membrane potential:
-
Glial Cells: Glial cells, such as astrocytes, play a significant role in regulating the extracellular ionic environment surrounding neurons, influencing the membrane potential and synaptic transmission.
-
Neuroactive Substances: Various neurotransmitters and neuromodulators influence the membrane potential by acting on different ion channels or receptors.
-
Metabolic Processes: Cellular metabolism and energy production can affect the activity of ion pumps, thereby indirectly impacting the membrane potential.
-
Pathological Conditions: Diseases and injuries can disrupt the normal ionic balance and cellular processes, leading to alterations in membrane potential and neuronal excitability. For example, epilepsy is characterized by abnormal, synchronized neuronal firing often associated with disrupted membrane potential regulation.
Measuring Membrane Potential: Patch Clamp Technique
The patch-clamp technique is a powerful electrophysiological method used to measure membrane potential and ionic currents in single neurons. This technique allows researchers to record the activity of individual ion channels and gain insights into the mechanisms underlying membrane potential fluctuations. Variations of the patch-clamp technique, such as the whole-cell patch clamp, enable researchers to manipulate the intracellular ionic composition and further investigate the role of specific ions in shaping the membrane potential.
The Significance of Understanding Membrane Potential
Understanding membrane potential and its regulation is fundamental to comprehending how the nervous system works. Disruptions in membrane potential can lead to various neurological disorders. Moreover, the principles of membrane potential and action potential generation underpin the development of new therapies and treatments for neurological diseases. For example, the development of drugs that target specific ion channels holds promise for treating conditions such as epilepsy, migraine, and chronic pain.
Research and Future Directions
Research on membrane potential is an active and evolving field. Scientists are continuously investigating the complex interplay of ion channels, receptors, and signaling pathways that regulate neuronal excitability. Advancements in imaging techniques and computational modeling are providing new insights into the spatiotemporal dynamics of membrane potential changes and their functional consequences. Further research into these areas is essential for developing more effective treatments for neurological and psychiatric disorders.
Conclusion: Depolarization - A Cornerstone of Neuronal Communication
This neuron is most depolarized at a specific mV – a value that depends upon numerous internal and external factors influencing the complex interplay of ion channels and pumps. This peak depolarization, however, remains a critical aspect of neuronal function, as it initiates the all-or-none action potential that underpins neuronal communication. The study of membrane potential and its intricacies remains a vibrant field, driving both fundamental understanding and the development of novel therapeutic strategies. Understanding the factors that influence this peak depolarization voltage is key to deciphering the complexities of neuronal signaling and the functioning of the nervous system as a whole. Further research and advancements in this field will undoubtedly lead to a deeper comprehension of the brain and its intricate mechanisms.
Latest Posts
Latest Posts
-
Research Suggests That The Sight Of A Weapon Can
May 05, 2025
-
For Each Item Below Indicate To Which Category
May 05, 2025
-
Del Is Buying A 250 000 Home
May 05, 2025
-
Declaring A Class Does Not Create Actual Objects
May 05, 2025
-
Select One Advantage Of Irr As A Capital Budget Method
May 05, 2025
Related Post
Thank you for visiting our website which covers about This Neuron Is Most Depolarized At Mv . We hope the information provided has been useful to you. Feel free to contact us if you have any questions or need further assistance. See you next time and don't miss to bookmark.